
International Journal of Earth Science and Geophysics
(ISSN: 2631-5033)
Volume 4, Issue 2
Research Article
DOI: 10.35840/2631-5033/1822
Effect of NZVI Treatment on the Physicochemical Properties of Pb-Contaminated Soil
Fuming Liu1,2,3, Shaoting Yi1,2, Rongmiao Xu2 and Shuping Yi1,2*
Table of Content
Figures
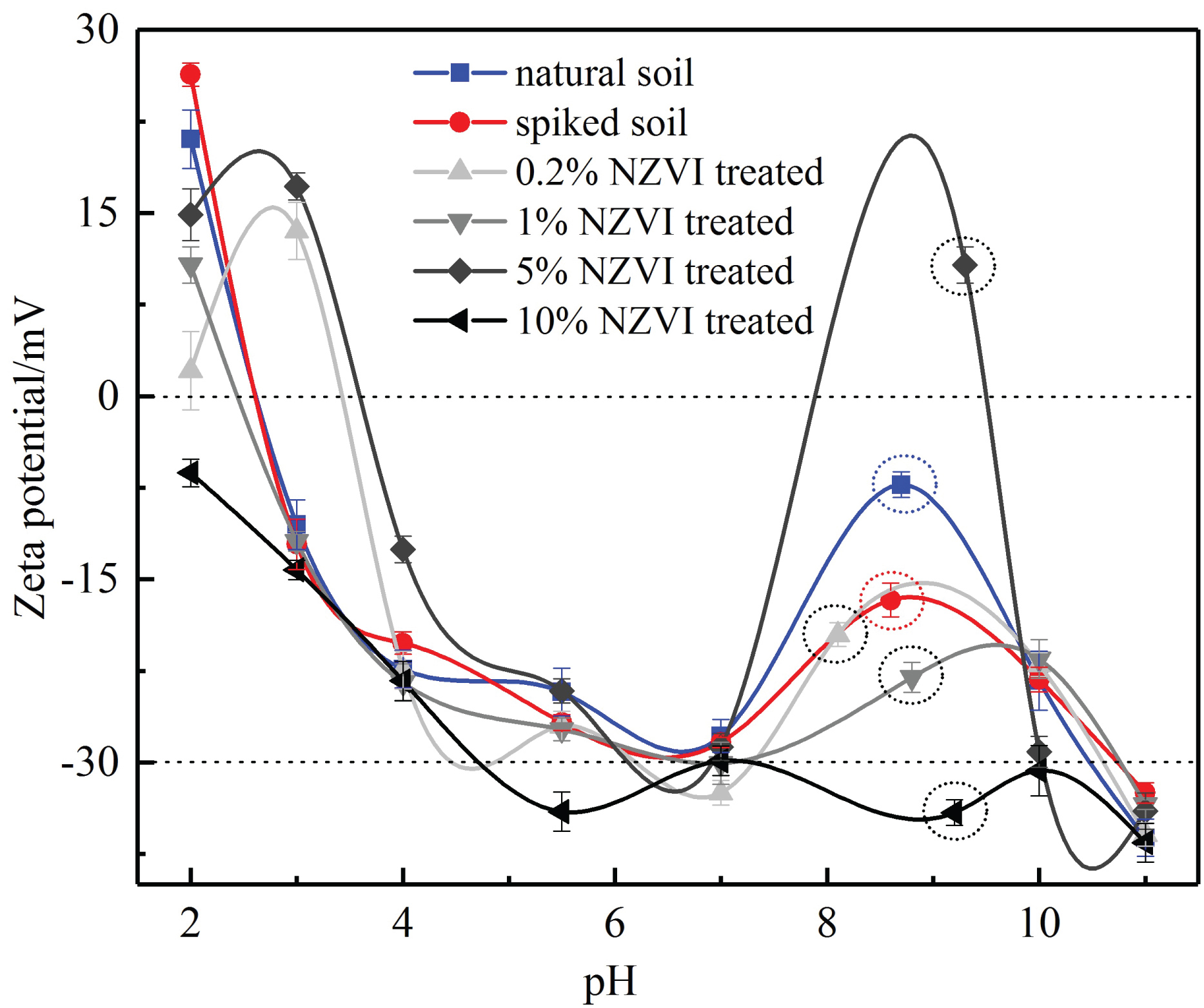
Figure 2: The ζ potential of soil specimens...
The ζ potential of soil specimens in water as a function of pH.

Figure 3: The changes in average soil...
The changes in average soil particle size versus BET surface area.
References
- Adeleye AS, JR Conway, K Garner, Y Huang, Y Su, et al. (2015) Engineered nanomaterials for water treatment and remediation: Costs, benefits, and applicability. Chemical Engineering Journal 286: 640-662.
- Liu Z, C Gu, Y Mao, Y Bian, Y Cheng, et al. (2015) Debromination of polybrominated diphenyl ethers by attapulgite-supported Fe/Ni bimetallic nanoparticles: Influencing factors, kinetics and mechanism. J Hazard Mater 298: 328-337.
- Shoemaker J, A Kney, S Mylon, A Merino, D Zhao (2010) Rapid destruction of perchlorate on ion exchange resin using stabilized, zero-valent iron nanoparticles (nZVI). World Environmental and Water Resources Congress.
- Yang X, X Shen, M Jing, R Liu, Y Lu, et al. (2014) Removal of heavy metals and dyes by supported nano zero-valent iron on barium ferrite microfibers. J Nanosci Nanotechnol 14: 5251-5257.
- De A, AK De, GS Panda, S Haldar (2017) Synthesis of zero valent iron nanoparticle and its application as a dephenolization agent for coke oven plant wastewater situated in West Bengal: India. Environmental Progress & Sustainable Energy.
- Liu GH, SN Zhu, Z Ye (2012) Reduction in the acute toxicity of explosive wastewater containing toxic nitroaromatic compounds by a nanoscale zerovalent iron pretreatment process. Water Air & Soil Pollution 223: 5049-5055.
- Diao ZH, XR Xu, D Jiang, LJ Kong, YX Sun, et al. (2016) Bentonite-supported nanoscale zero-valent iron/persulfate system for the simultaneous removal of Cr(VI) and phenol from aqueous solutions. Chemical Engineering Journal 302: 213-222.
- Li J, L Zhang, C Guo, M Shi, J Li, et al. (2017) Removal of Cr(VI) by sewage sludge based activated carbons impregnated with nanoscale zero-valent iron. Journal of Nanoscience & Nanotechnology 17: 6936-6941.
- Fageeh O, SA Al-Thabaiti, MA Salam (2017) Kinetics and thermodynamics studies of the nitrate reduction using zero valent iron nanoparticles supported on nanographene. Nanoscience & Nanotechnology Letters 9: 338-347.
- Harada T, T Yatagai, Y Kawase (2016) Hydroxyl radical generation linked with iron dissolution and dissolved oxygen consumption in zero-valent iron wastewater treatment process. Chemical Engineering Journal 303: 611-620.
- Noubactep C (2008) A critical review on the process of contaminant removal in Fe0-H2O systems. Environmental Technology 29: 909-920.
- Lefevre E, N Bossa, MR Wiesner, CK Gunsch (2016) A review of the environmental implications of in situ remediation by nanoscale zero valent iron (nZVI): Behavior, transport and impacts on microbial communities. Science of the Total Environment 565: 889-901.
- Fan D, DM O'Carroll, DW Elliott, Z Xiong, PG Tratnyek, et al. (2016) Selectivity of nano zerovalent iron in in situ chemical reduction: Challenges and improvements. Remediation Journal 26: 27-40.
- Du YJ, RD Fan, KR Reddy, SY Liu, YL Yang (2015) Impacts of presence of lead contamination in clayey soil-calcium bentonite cutoff wall backfills. Applied Clay Science 108: 111-122.
- Fan RD, SY Liu, YJ Du, KR Reddy, YL Yang (2016) Impacts of presence of lead contamination on settling behavior and microstructure of clayey soil - calcium bentonite blends. Applied Clay Science 142.
- Yang YY, YS Xu, SL Shen, Y Yuan, ZY Yin (2015) Mining-induced geo-hazards with environmental protection measures in Yunnan, China: an overview. Bulletin of Engineering Geology and the Environment 74: 141-150.
- Huang Y, P Jin (2017) Impact of human interventions on coastal and marine geological hazards: A review. Bulletin of Engineering Geology and the Environment 77: 1081-1090.
- Nasehi SA, A Uromeihy, MR Nikudel, A Morsali (2016) Use of nanoscale zero-valent iron and nanoscale hydrated lime to improve geotechnical properties of gas oil contaminated clay: A comparative study. Environmental Earth Sciences 75: 733.
- Fu F, DD Dionysiou, H Liu (2014) The use of zero-valent iron for groundwater remediation and wastewater treatment: A review. J Hazard Mater 267: 194-205.
- O'Carroll D, B Sleep, M Krol, H Boparai, C Kocur (2013) Nanoscale zero valent iron and bimetallic particles for contaminated site remediation. Advances in Water Resources 51: 104-122.
- Indraratna B, IW Redana (1998) Laboratory determination of smear zone due to vertical drain installation. Journal of Geotechnical and Geoenvironmental Engineering 124: 180-184.
- Mar Gil‐Díaz M, A Pérez‐Sanz, M Ángeles Vicente, M Carmen Lobo (2014) Immobilisation of Pb and Zn in soils using stabilised zero‐valent iron nanoparticles: Effects on soil properties. CLEAN-Soil, Air, Water 42: 1776-1784.
- Bae S, K Hanna (2015) Reactivity of nanoscale zero-valent iron in unbuffered systems: Effect of pH and Fe(II) Dissolution. Environmental Science & Technology 49: 10536-10543.
- Sunil BM, S Nayak, S Shrihari (2006) Effect of pH on the geotechnical properties of laterite. Engineering Geology 85: 197-203.
- Gupta V, I Ahmed (2007) The effect of pH of water and mineralogical properties on the slake durability (degradability) of different rocks from the Lesser Himalaya, India. Engineering Geology 95: 79-87.
- Ghobadi MH, Y Abdilor, R Babazadeh (2014) Stabilization of clay soils using lime and effect of pH variations on shear strength parameters. Bulletin of Engineering Geology and the Environment 73: 611-619.
- Liu X, P Hicher, B Muresan, N Saiyouri, PY Hicher (2016) Heavy metal retention properties of kaolin and bentonite in a wide range of concentration and different pH conditions. Applied Clay Science 119: 365-374.
- Spagnoli G, D Rubinos, H Stanjek, T Fernández-Steeger, M Feinendegen, et al. (2012) Undrained shear strength of clays as modified by pH variations. Bulletin of Engineering Geology and the Environment 71: 135-148.
- Sipos P, T Németh, I Mohai, I Dódony (2005) Effect of soil composition on adsorption of lead as reflected by a study on a natural forest soil profile. Geoderma 124: 363-374.
- Li S, W Wang, Y Liu, Wx Zhang (2014) Zero-valent iron nanoparticles (nZVI) for the treatment of smelting wastewater: A pilot-scale demonstration. Chemical Engineering Journal 254: 115-123.
- Yukselen Y, A Kaya (2003) Zeta potential of kaolinite in the presence of alkali, alkaline earth and hydrolyzable metal ions. Water Air & Soil Pollution 145: 155-168.
- Vallar S, D Houivet, JE Fallah, D Kervadec, JM Haussonne (1999) Oxide slurries stability and powders dispersion: optimization with zeta potential and rheological measurements. Journal of the European Ceramic Society 19: 1017-1021.
- Lu N, M Khorshidi (2015) Mechanisms for soil-water retention and hysteresis at high suction range. Journal of Geotechnical and Geoenvironmental Engineering 141: 04015032.
- Ding H, Y Wu, B Zou, Q Lou, W Zhang, et al. (2016) Simultaneous removal and degradation characteristics of sulfonamide, tetracycline, and quinolone antibiotics by laccase-mediated oxidation coupled with soil adsorption. Journal of Hazardous Materials 307: 350-358.
- Yukselen-Aksoy Y, A Kaya (2011) A study of factors affecting on the zeta potential of kaolinite and quartz powder. Environmental Earth Sciences 62: 697-705.
- Bui QB, JC Morel, S Hans, P Walker (2014) Effect of moisture content on the mechanical characteristics of rammed earth. Construction & Building Materials 54: 163-169.
- Li ZY, RK Xu, JY Li, ZN Hong (2016) Effect of clay colloids on the zeta potential of Fe/Al oxide-coated quartz: A streaming potential study. Journal of Soils & Sediments 16: 2676-2686.
- Zulfiqar W, MA Iqbal, MK Butt (2017) Pb2+ ions mobility perturbation by iron particles during electrokinetic remediation of contaminated soil. Chemosphere 169: 257-261.
- Tholkappiyan R, K Vishista (2015) Synthesis, structural, magnetic and xps studies of garnet type-dysprosium iron oxides by glycine-assisted combustion method. Nanoscience & Nanotechnology Letters 7: 469-475.
- Chen X, X Dou, D Mohan, CU Pittman, M Hu, et al. (2016) Effects of surface iron hydroxyl group site densities on arsenate adsorption by iron oxide nanocomposites. Nanoscience & Nanotechnology Letters 8: 1020-1027.
Author Details
Fuming Liu1,2,3, Shaoting Yi1,2, Rongmiao Xu2 and Shuping Yi1,2*
1Shenzhen Key Laboratory of Soil and Groundwater Pollution Control, China
2School of Environmental Science and Engineering, Southern University of Science and Technology, China
3Department of Civil and Environmental Engineering, Faculty of Science and Technology, University of Macau, China
Corresponding author
Dr. Shuping Yi, Research Associate Professor, Shenzhen Key Laboratory of Soil and Groundwater Pollution Control, Shenzhen, China; School of Environmental Science and Engineering, Southern University of Science and Technology, Shenzhen, China.
Accepted: November 17, 2018 | Published Online: November 19, 2018
Citation: Liu F, Yi S, Xu R, Yi S (2018) Effect of NZVI Treatment on the Physicochemical Properties of Pb-Contaminated Soil. Int J Earth Sci Geophys 4:022.
Copyright: © 2018 Liu F, et al. This is an open-access article distributed under the terms of the Creative Commons Attribution License, which permits unrestricted use, distribution, and reproduction in any medium, provided the original author and source are credited.
Abstract
Nanoscale Zero Valent Iron (NZVI) is the most widely used engineering nanomaterials in the remediation of contaminated site. This study investigated the effect of NZVI treatment on the physicochemical properties of Lead (Pb)-contaminated soil to improve the understanding of the soil improvement. The changes of soil physicochemical properties were investigated using the concentration range of 0.2%, 1%, 5%, and 10% NZVI g/g dry soil. Results showed that the introduction of NZVI to the Pb-contaminated soil resulted in substantial changes in the surface physicochemical properties. The ferric and ferrous ions, and the lead ions were released at the same time from the corrosion of NZVI precipitation, and from the surface of soil particles, respectively, amongst which, part of exchangeable lead ions were reduced to Pb(I) and/or Pb0. A general change in the soil's electrical conductivity, BET surface area, soil buffering capacity, and soil's particle size was confirmed. A conclusion was drawn that the mass transfer of NZVI and its induced precipitation of Fe/Pb ions made a great contribution to the changes of soil physicochemical properties. This study could make for the application of nanomaterials in remediation of contaminated sites, especially for the brownfield remediation and backfill usage after the contaminated soil was remediated.
Keywords
Contaminated soil, Nanomaterials, Physicochemical characterization, Soil improvement
Introduction
Nanoscale Zero Valent Iron (NZVI) and other iron-mediated nanoparticles are the most widely used engineering materials in the remediation of contaminated site in the United States and the Europe, especially in the in-situ remediation application as they are cost-effective [1]. NZVI is a versatile nanomaterial for chemical removal of various organic and inorganic contaminants such as halogenated organic compounds (HOCs) [2], perchlorate [3], dyes [4], phenol [5], nitroaromatic compounds (NACs) [6], arsenic [7], heavy metals [8], and nitrate [9]. The removal mechanism of contaminants by NZVI is dominated by adsorption, reduction, oxidation and (co)precipitation. Metallic iron (Fe0) is an effective reductant with standard redox potential (E0 = -0.44V). Variety of contaminants can be easily adsorbed onto the surface of NZVI due to its high specific surface area and surface activity induced by its nanoscale particle size. The adsorbed oxidative contaminants such as Cr(VI) could transform into non-toxic or less toxic species via direct transfer of electrons from NZVI to the contaminants. The NZVI acts as an electron donor and simultaneously get oxidized to Fe(Ⅱ) and/or Fe(Ⅲ) oxidation states:
Both organic compounds and inorganic compounds can act as electron acceptor and then be reduced. Though this reductive reaction is very effective, its capable of mineralization is limited for organic and inorganic compounds [10]. Additionally, the Dissolved Oxygen (DO) can also accept electron to produce H2O2:
The hydroxyl radicals (·OH) induced by the combination of the produced H2O2 and Fe(Ⅱ) (known as Fenton reaction or Fenton-like reaction), which possess strong and non-selective oxidizing capability of mineralization of most organic compounds:
The oxidative ferric iron ions can be reduced by zero-valent iron to provide more ferrous, and more associated hydroxyl radicals to accelerate the degradation of organic compounds:
The established iron redox cycling promotes the generation of ferric and ferrous ions. Meanwhile, iron oxide or hydroxide deposit on the surface of NZVI as the erosion of NZVI. Iron oxides or hydroxides are capable of adsorbing contaminants onto the surface of NZVI [11]. Under particular circumstances, these hydroxides could transform into variety of less adsorptive oxides such as FeO, FeOOH via dehydration and crystallization. On the other hand, the deuterogenic ferric and ferrous temporarily produced in the iron redox system are only stable under specific conditions, they are easily oxidized to insoluble iron species such as Fe3O4 (maghemite), Fe3O4 (magnetite), FeOOH (lepidocrocite), or goethite [12]. In this regarding, part of resistant contaminants can be removed through (co)precipitation.
It was reported that a great market demanding for the application of NZVI in contaminated sites throughout Asian countries, especially in China [13]. The application of NZVI in remediation receives a greater motive for the brownfields as their expensive and limited land resources for the construction of commercial and residential purposes. However, it has been demonstrated that the increasing use of NZVI for in-situ remediation of contaminated site is raised some challenges due to its potential adverse impacts on indigenous microbial communities and ecosystem functioning [12]. Worse still, few is known regarding the effect of NZVI on the soil geotechnical properties.
It should be noted that the soil geotechnical properties are crucial for civil engineering constructions. Both the contaminants and NZVI introduced to the subsurface are exotic substances for the pristine soil. The changes of soil physicochemical properties derived from contamination and remediation may undermine the foundation, leading to the occurrence of engineering accidents ultimately. For instance, chemical contaminants, such as lead ions, generally give negative effects on the soil's geotechnical properties. The liquid limit, specific surface area, pH and compression index of soil decreased as the increasing Pb concentration in the clayey soil used as cutoff wall backfills [14]. Besides, the hydraulic conductivity even exceeded the typical regulatory limit of 10-9 m/s when the Pb concentration exceeded a threshold value of 120 mmol/L. In addition to that, lead concentration has a significant impact on the settling behaviour and sediment volume of kaolin-bentonite blends, attributing to the changes in particle interaction varied with ionic concentration and pH [15].
The changes of soil's geotechnical properties by chemical contamination are always incarnated in the alterations of soil's physicochemical properties. Contamination and remediation of soil and groundwater can cause serious geo-hazards such as collapse, landslide, debris flow, sinkhole collapse, and earth fissure [16,17]. When the contaminated soils are remedied using chemical methods, e.g. NZVI involved and In-Situ Chemical Oxidation, the soil's physicochemical properties will change when conducting the injection of remedial reagents to remove contaminants in soil, ultimately affect the reliability of the treated soil when used as foundation materials.
However, few studies focus on the chemical remediation derived geoenvironmental concerns. Nasehi, et al. [18] investigated the effects of NZVI and Nanoscale Hydrated Lime (NHL) on the geotechnical characteristics of a clayey soil contaminated by gas oil, in which they suggested that the increase of the cohesion and internal friction angle of the samples treated with NZVI is ascribed to the attractive force between the NZVI and surface of clay particles and frictional characteristic of NZVI powder [18]. As a matter of fact, the NZVI is a versatile nanomaterial that can efficiently remove these contaminants involved in not only organic contaminants, but also inorganic contaminants such as arsenic, heavy metals, and nitrate [19]. The predominant removal mechanism for organic contaminants is mineralization derived by Advanced Oxidation Process (AOP), and is a combination of reduction, while adsorption, oxidation and precipitation is the predominant removal mechanism for the most common inorganic contaminants [20]. Therefore, the behaviour of NZVI in the soil improvement should be investigated, especially for the removal of inorganic contaminants in which different removal mechanisms are involved.
This study presents an investigation of the effect of NZVI treatment on the Pb-contaminated soil particles. The aims of this study were to characterize the changes of soil's physicochemical properties so that to improve the understanding of soil improvement resulted by the NZVI treatment. These results can facilitate the application of nanomaterials in the remediation of contaminated site, especially for the remediation of brownfield and the contaminated site in which remedied soil will be reused as backfill.
Methodology
Soil preparation
The soil used in this study was sampled from a construction site in Areia Preta, Macau. X-ray Diffraction (XRD) analysis indicated that they mainly composed of quartz (SiO2), laumontite (Ca4(Al8Si16O48(H2O)18), and greenalite (Fe6Si4O10(H2O)18). The obtained soil was successively slurried, sieved through a No. 40 sieve (0.425 mm opening) and adjusted the water content maintained at approximately 125% (the double of its liquid limit). Then the soil slurry was spiked at the Pb concentration of 400 mg/kg (dry soil mass, Lead Nitrate (Pb(NO3)2)) and aged for two weeks. After that, the aged slurry was preconsolidated in a soil sampler maker to simulate the subsurface pressure with an overburden pressure maintained at approximately 200 kPa. A more detailed description of the soils sample maker in this study area can be found in Indraratna, et al. [21]. When the change of the consolidation settlement was stable, the soil specimens were elaborately unload and trimmed at the height of 84 mm and diameter of 38 mm. For the preparation of NZVI treated soil specimens, a preconcerted amount of NZVI the dose of 0.2%, 1%, 5%, and 10% (mass ratio of NZVI to the spiked dry soil) were added respectively to the aged Pb-spiked slurry and were vividly agitated prior to preconsolidation. The NZVI supplied by Shanghai Xiangtian Nano Materials co., LTD was used as received. The claimed average diameter of NZVI was about 50 nm, with the specific area of 30 m2/g.
Physicochemical characterization of soil particles
In this study, the acid buffering capacity and ζ potential of soil particles were determined. Soil buffer capacity was determined by titration modified by Gil, et al. [22]. In brief, 10 g of dry soil were placed in a glass beaker that contained 200 mL of deionised water (50 g/L), mixed and ultrasound treated for 10 min. Then, 100 uL of 5 mol/L Hydrochloride acid (HCl) was added at an interval time of 5 min, until the pH value reach approximately 2. The ζ potential electrical conductivity of soil specimens were measured using ζ potential Analyzer (Brookhaven BI-200 SM) at the concentration of 0.2 g/L, with deionized water as the dispersion.
Moreover, the particle size, pore size, and surface area of the soil specimens was analysed using a BET Micromeritics Tristar 300 Surface Analyzer. The changes of NZVI and Lead ions were used to characterize using X-ray photoelectron spectroscopy (XPS).
Results and Discussion
Soil buffering capacity
Buffering capacity is the capability of the soil to resist the changes of pH value and the pH value of the reaction system is regarded as one of the most important factors that controlling the kinetic rate constants of contaminants removal by NZVI. For instance, the decrease of pH value resulted in an increase of 4-nitrophenol (4-NP) and a decrease of lead removal [23]. Furthermore, the changes of pH value will affect the geotechnical properties of soil by influencing the chemical characteristics of soil, including mineralogical composition, ion exchange, ions adsorption competition, and surface precipitation [24-27]. For instance, it is found that the undrained shear strength for clays increased considerably if the pore fluid had a high or a low pH value [28]. Hence, it is significant to reveal the soil buffer capacity of NZVI treated soil.
Figure 1 shows the buffering capacity of natural soil, Pb-spiked soil and NZVI treated soils. Generally, the pH value of all soil specimens dropped fleetly first from 9.0 to 5.5, then decreased tardily when pH value is less than 5.5. This result suggesting that the soil used in this experiment endows a relatively poor buffering capacity. A feeble change regarding the pH value from 5.5 to 2.0 could be noted for the specimens after the introduction of Lead Nitrate and NZVI. For the Pb contaminated soil specimen, the decrease of its pH buffering capacity could be ascribed to the partly decomposition of organics by the lead [29]. As a comparison, the NZVI treated soils present a relative better buffering capacity in particular at less treatment doses (0.2% and 1% amount of NZVI). The NZVI treatment led to a limited improvement of soil buffering capacity could be principally attributed to the equilibrium between the decrease of organic compounds, increase of derived iron minerals, and the change of soil components (e. g. anions, cations) [30].
Zeta potential
Zeta potential is the electric potential in the interfacial double layer at the location of the slipping plane relative to a point in the bulk fluid away from the interface. ζ potential of soil particles is a good pointer of their electrical potentials: The higher the ζ potential, the higher the surface potential of charged soil particle as well as the higher stability of the aqueous suspensions of particles [31]. ζ potential of particles is highly dependent on both the pH and their density [32]. The analysis of the ζ potential allows us to investigate the removal characteristics of contaminants and the effect of moisture content on the mechanical characteristics of soil [33,34]. For instance, the cohesion of clayey soil material was provided by both the electrostatic forces between particles and cementing of clay particles. According to the Gouy-Chapman model, as the concentration of ions or valence of ions increases, the thickness of diffuse electrical double layer decreases resulting a lower ζ potential [35]. Bui, et al. [36] suggested that the increase of the concentration and of valence of the cations will not only induce a low thickness of the double layers but also loses its cohesion [36].
Variations in the ζ potentials of natural soil, Pb-spiked soil and NZVI treated soil are given in Figure 2. Generally, the natural clay soil and Pb-spiked soil present negatively charged except that in the very sour conditions (pH < 2.6). The ζ potential of NZVI treated soil specimens fluctuates acutely with the increase of pH value. Although the ζ potentials values of NZVI treated soil specimens are different, they trend with pH is similar. As the increase of pH value in the solution, the ζ potential of NZVI treated soil mostly changed from positively charged to negatively charged, then converged to approximately -30 mV at the neutral pH value. After that, the ζ potential of the soil specimens fluctuated clearly and converged again to approximately -33 mV at the pH value of 11. The corrosion of NZVI induced derived iron minerals on soil and the overlapping of the diffuse layers of electrical double layers were responsible for the changes in ζ potential of the soil [37]. Note that 10% NZVI treated soil does not give a point of zero charge with pH value ranges from 2 to 11; however, the 5% NZVI treated soil gives two apparent PZCs (Point of Zero Charge). Because a mineral can only get only one PZC [31], the appearance of two apparent PZCs can be explained with the hybrid precipitation of metal ions in alkalescent condition:
The abundant hydroxyl generated in the redox reactions (3) and (4) provided an alkalescent condition for the hybrid precipitation. It should be noted that the changes in the soil's ζ potential is the complex result of the changes of particle's surface physicochemical properties, in which the hybrid precipitation of Pb/Fe metal ions is highly associated, and vice versa. That is, variations in the ζ potentials of natural soil, Pb-spiked soil and NZVI treated soil mean the occurrence of the hybrid precipitation of Pb/Fe metal ions on the soil particle.
BET surface features
The microstructure of soil specimens was characterized by BET surface area and average particle size, as shown in Figure 3. Results indicate that a slight increase in the BET surface area of soil specimen along with a tiny decrease in the average particle size was recorded as the introduction of Lead Nitrate. This phenomenon suggests that the Lead Nitrate is corrosive so that part of natural organic matters adsorbed on the soil particles [38], or the bonded soil particles may be decomposed, and lead to a decreased in the average soil particle size. For the NZVI treated soil specimens, a gradual decrease in the average particle size was found as the increase of added nanoscale NZVI except for the 10% NZVI treated soil specimen. Accordingly, the BET surface area of NZVI treated soil specimens increased by degrees. The decrease in average particle size can be ascribed to the increase of NZVI aggregates in nanoscale in the soil in spite of its corrosion. However, the 10% NZVI treated soil specimen shown negative growth in average particle size compared with the 5% NZVI treated soil specimen. It is speculated that this abnormal phenomenon was derived by the superior cementation (precipitation of Pb/Fe metal ions) of soil particles by which to achieve a rapid increase of soil particles in larger size. On the other hand, the higher treatment dose of NZVI gave rise to an increased in the grain contact probability in the soil slurry, exacerbating the precipitates-enrichment effect to form iron oxides in a larger size, ultimately level up the average soil particle size.
XPS
The soil surface chemical property was further characterized by X-ray Photoelectron Spectroscopy (XPS) with Fe and Pb elements focused. As showed in Figures 4, 2p3/2 peaks of Fe in samples were stronger than 2p1/2 peaks, the 10% NZVI treated soil presented stronger peaks (both of Fe 2p3/2 and Fe 2P1/2) than other soil. Natural soil and Pb polluted soil both have two peaks that weaker peak was Fe(Ⅲ), while stronger was Fe(Ⅱ). The Fe(Ⅱ) and Fe(Ⅲ) peak of Fe 2P1/2 with a narrow binding energy interval was because of more complicated component that impact Fe binding energy. The Fe(Ⅱ) and Fe(Ⅲ)can be credited to the derived iron mineral crystals of ferrosilite FeSiO3, hematite Fe2O3, goethite FeOOH, and magnetite Fe3O4, respectively [39]. Compared with natural soil and Pb polluted soil, the XPS spectrum of 10% NZVI treated soil presented slightly shifting indicating that NZVI changed surface atom chemical bond structure of soil. XPS techniques only characterize the surface several nanometers of material and Nano-zero-valent iron was easily oxidized resulting in the absence of zero-valent iron peak [40].
The availability of Pb in soil specimens analyzed after applying the Modified Sequential Extraction Procedure (MSEP) suggested that the lead in more easily available fractions (in the exchangeable and carbonate-bound fractions) was evidently decreased by the introduction of NZVI (the MSEP result doesn't present in this paper). However, the XPS spectrum of natural soil indicates that almost no Pb0 on the surface of natural soil, indicating that the (co)precipitation was the primary mechanism for the stabilization of Pb as the NZVI treatment. The two peaks were Pb 4f7/2 and 4f5/2, respectively. As showed in Figure 5 that the peak of Pb 4f7/2 was stronger than Pb 4f5/2 were because it's a main characteristic peak. Pb2+ lost two electrons and became bigger binding energy. XPS techniques only characterize the surface several nanometers of material and the Pb of surface of 10% NZVI treated soil was easily oxidized resulting in that there no zero-valent Pb peaks.
Conclusion
Using nanomaterials to remedy the contaminated soil and groundwater is one of well-established technologies that has been widely implemented in both the laboratory test and engineering project. This paper studied the physicochemical properties of Pb-contaminated soil treated by NZVI and attempted to gain the understanding the NZVI derived soil improvement. The variations of the soil buffer capacity, zeta potential, BET surface feature, and surface chemical property of soil particles were analyzed. Results indicated that the introduction of NZVI to the Pb-contaminated soil resulted in substantial changes in the surface physicochemical properties of soil particles. The substantial changes of soil surface physicochemical properties as a result of the precipitation of Fe/Pb hydrated oxides was presumably contributed to the primary mechanism for the NZVI in the soil improvement. More works should be done to reveal its mechanism at a deeper level for the changes of physicochemical properties associated with the geotechnical properties of soil, consequently promoting the application of highly reactive materials in the remediation of contaminated site, especially for the remediation of brownfield and the contaminated site in which the remedied soil is used as backfill.
Acknowledgements
The authors wish to thank financial support from the Shenzhen Peacock Plan (KQTD2016022619584022), and Shenzhen Key Laboratory of Soil and Groundwater Pollution Control, South University of Science and Technology of China.