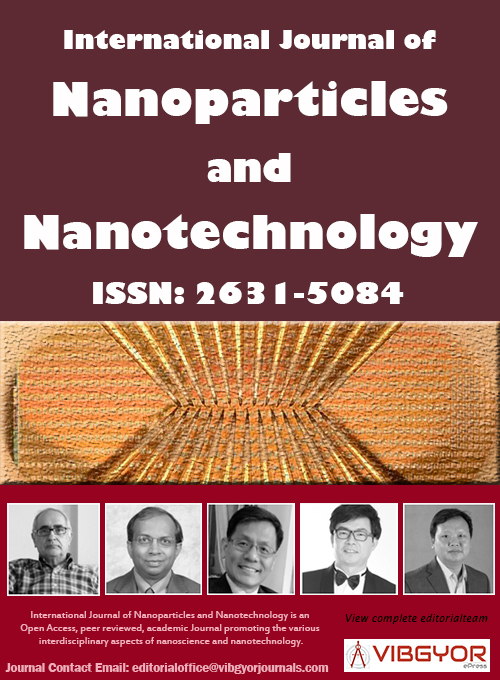
International Journal of Nanoparticles and Nanotechnology
(ISSN: 2631-5084)
Volume 3, Issue 1
Research Article
DOI: 10.35840/2631-5084/5511
Facile Synthesis of Polyaniline-Prussian Blue Composite for High-Performance Supercapacitors
Xueying Yang1, Jijue Li1, Xinkun Song1, Yingzhao He1, Xiaoxiao Du1, Yongjin Zou1,2* and Haitao Zhang1,2
Table of Content
Figures
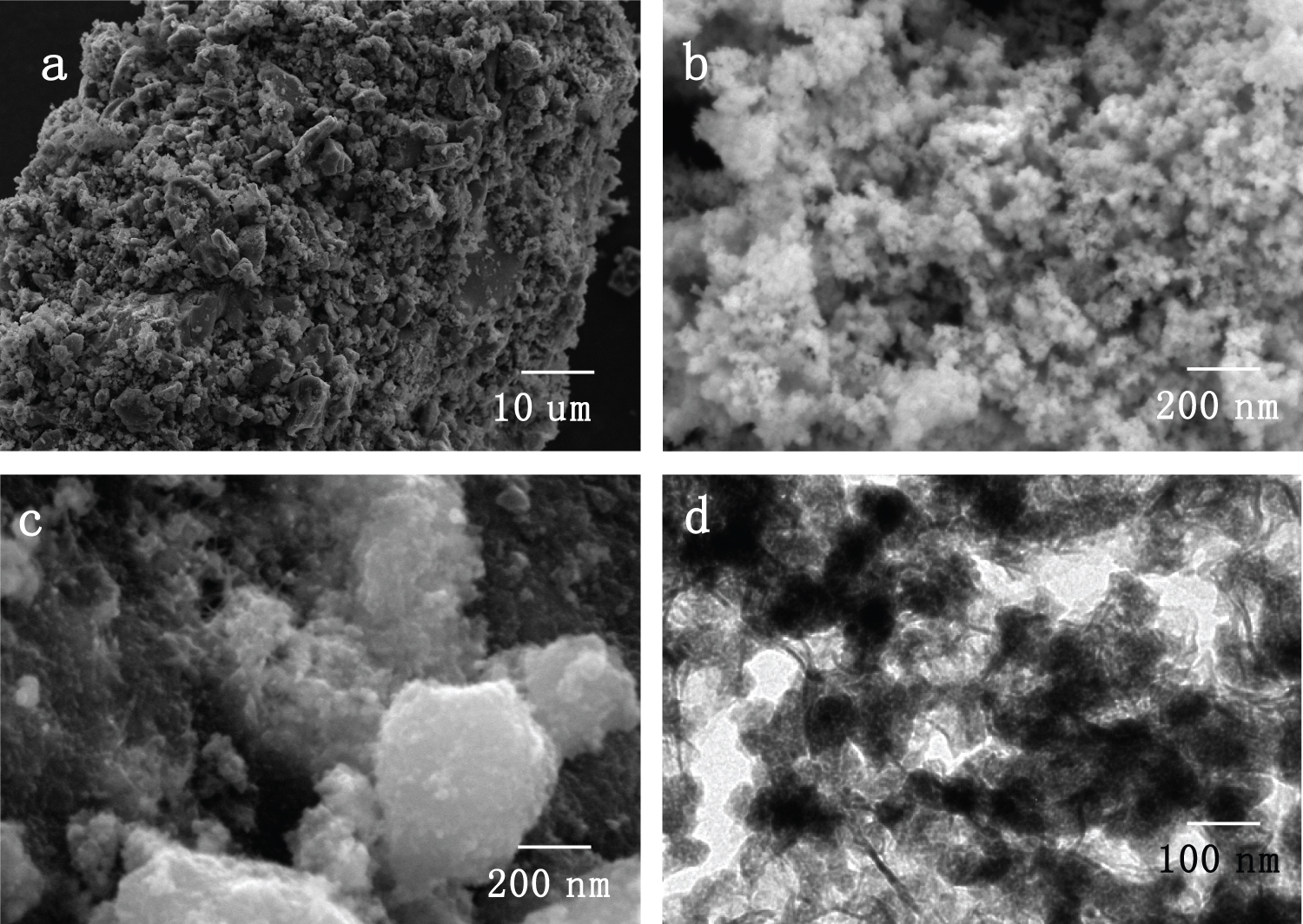
Figure 1: SEM images of a) PB; b)...
SEM images of a) PB; b) PANI; c) PANI-PB; TEM image of d) PANI-PB.
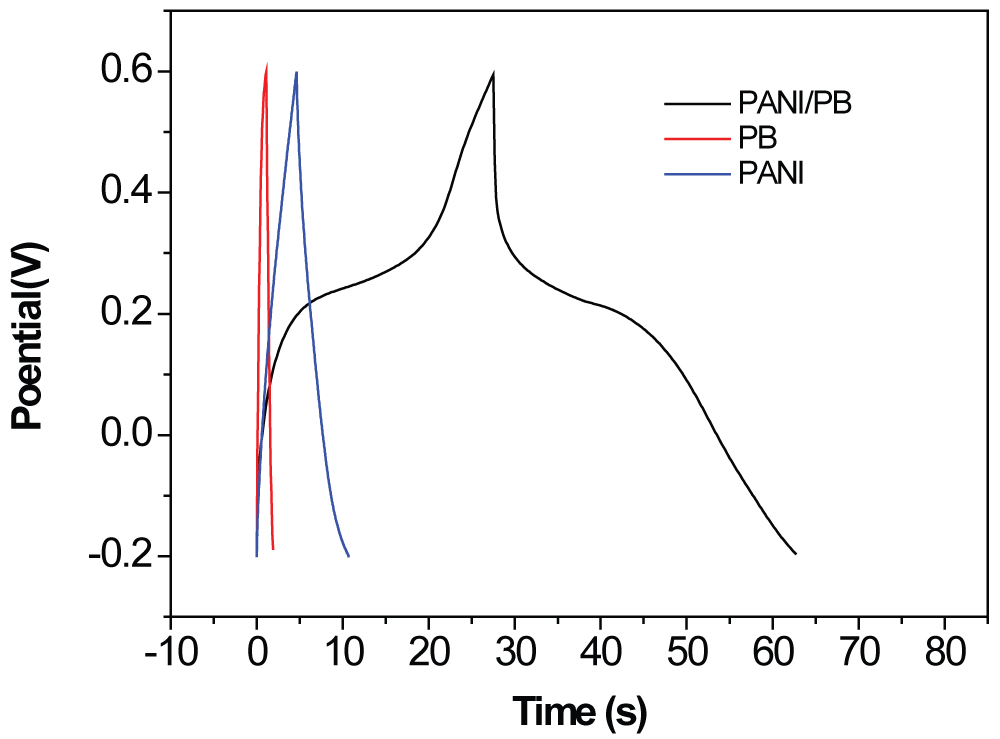
Figure 4: GCD curves for the PB, PANI...
GCD curves for the PB, PANI, and PANI-PB composite electrodes at 5 A g-1.
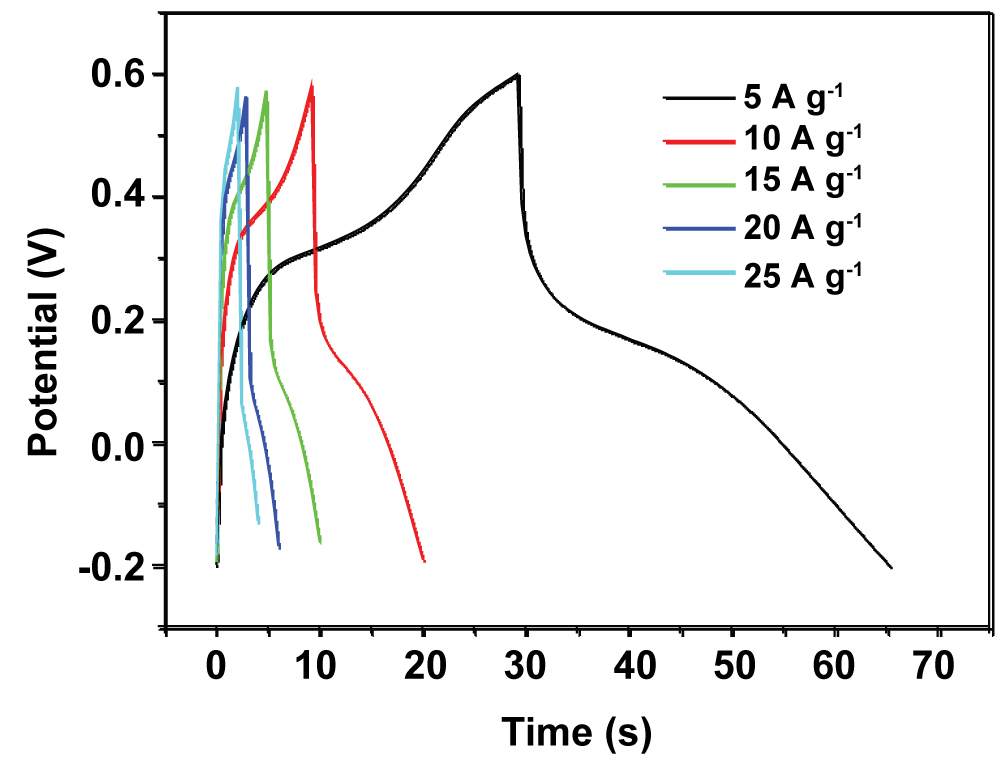
Figure 5: Charge/discharge curves for the PANI-PB...
Charge/discharge curves for the PANI-PB electrode at current densities of 5-25 A g-1.
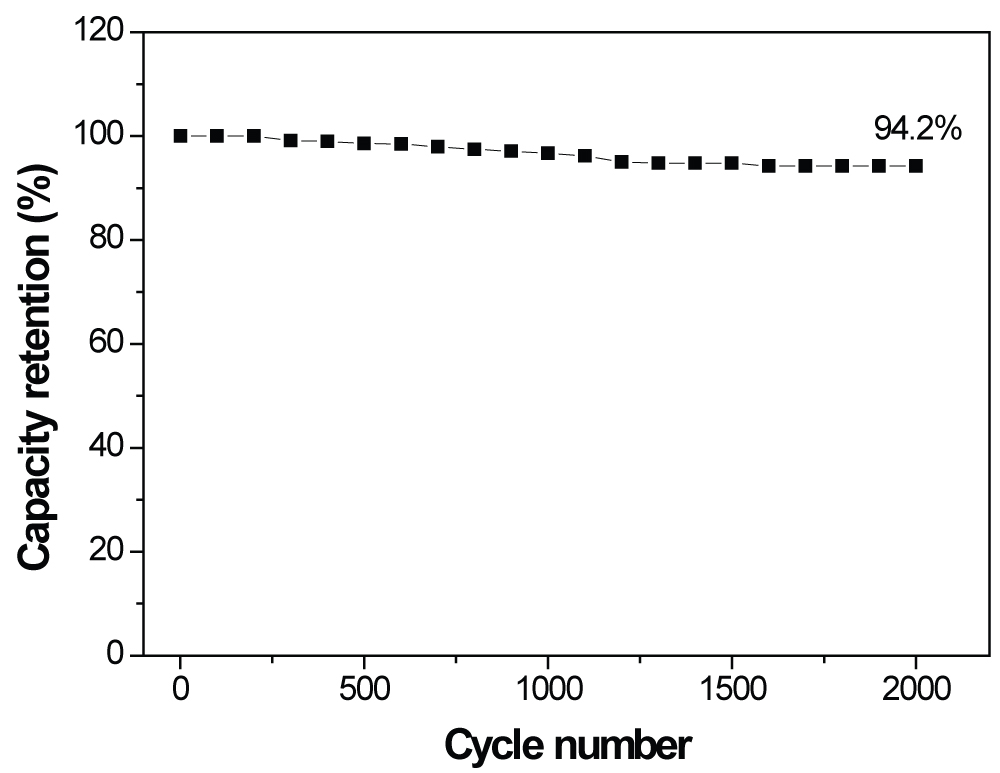
Figure 6: Capacity retention of the PANI-PB electrode...
Capacity retention of the PANI-PB electrode over 2000 cycles at a current density of 5 A g-1.
References
- Singh D, Murthy JY, Fisher TS (2011) Mechanism of Thermal Conductivity Reduction in Few-Layer Graphene. Journal of Applied Physics 110: 044317.
- Balandin AA, Ghosh S, Bao W, Calizo I, Teweldebrhan D, et al. (2008) Superior Thermal Conductivity of Single-Layer Graphene. Nano Lett 8: 902-907.
- Chang C, W-Q Han, Zettl A (2005) Thermal conductivity of B-C-N and BN nanotubes. Journal of Vacuum Science & Technology B, Nanotechnology and Microelectronics: Materials, Processing, Measurement, and Phenomena 23: 1883-1886.
- Golberg D, Bando Y, Huang Y, Terao T, Mitome M, et al. (2010) Boron Nitride Nanotubes and Nanosheets. AZO Nano 4: 2979-2993.
- Chen Y, FitzGerald J, Williams JS, Bulcock S (1999) Synthesis of boron nitride nanotubes at low temperatures using reactive ball milling. Chemical Physics Letters 299: 260-264.
- Yu J, Chen Y, Wuhrer R, Liu Z, Ringer SP (2005) In Situ Formation of BN Nanotubes during Nitriding Reactions. Chem Mater 17: 5172-5176.
- Loiseau A, Willaime F, Demoncy N, Hug G, Pascard H (1996) Boron nitride nanotubes with reduced numbers of layers synthesized by arc discharge. Phys Rev Lett 76: 4737-4740.
- Terrones M, Hsu WK, Terrones H, Zhang JP, Ramos S, et al. (1996) Metal particle catalysed production of nanoscale BN structures. Chemical Physics Letters 259: 568-573.
- Lourie OR, Jones CR, Bartlett BM, Gibbons PC, Ruoff RS, et al. (2000) CVD Growth of Boron Nitride Nanotubes. Chem Mater 12: 1808-1810.
- RZ Ma, Y Bando, T Sato, K Kurashima (2001) "Growth, Morphology, and Structure of Boron Nitride Nanotubes". Chem Mater 13: 2965-2971.
- JS Wang, VK Kayastha, YK Yap, ZY Fan, JG Lu, et al. (2005) Low Temperature Growth of Boron Nitride Nanotubes on Substrates. Nano Lett 5: 2528-2532.
- Golberg D, Bando Y, Eremets M, Takemura K, Kurashima K, et al. (1996) Nanotubes in boron nitride laser heated at high pressure". Appl Phys Lett 69: 2045-2047.
- Chen Y, Conway M, Williams JS, Zou J (2002) Large-quantity production of high-yield boron nitride nanotubes. Journal of Materials Research 17: 1896-1899.
- Golberg D, Bando Y, Han W, Kurashima K, Sato T (1999) Single-walled B-doped carbon, B/N-doped carbon and BN nanotubes synthesized from single-walled carbon nanotubes through a substitution reaction. Chemical Physics Letters 308: 337-342.
- Terauchi M, Tanaka M, Suzuki K, Ogino A, Kimura K (2000) Production of zigzag-type BN nanotubes and BN cones by thermal annealing. Chemical Physics Letters 324: 359-364.
- Edwards RS, Coleman KS (2013) Graphene synthesis: relationship to applications. Nanoscale 5: 38-51.
- http://proedgewire.com/graphite-graphene-intel/china-sets-access-standards-for-its-graphite-industry/.
- Chakrabarti A, Lu J, Skrabutenas JC, Xu T, Xiao Z, et al. (2011) Conversion of CarbonDioxide to Few-layer Graphene. J Mater Chem 21: 9491-9493.
- Park KT, Nersisyan HH, Chun BS, Lee JH (2011) Preparation of porous zirconium microspheres by magnesio thermic reduction and their microstructural characteristics. Journal of Materials Research 26: 2117-2122.
- (2015) Technical report submitted to NASA for contract NNX12CA43C.
- Khanam PN, Ponnamma D, AL-Madeed MA (2015) Electrical Properties of Graphene Polymer Nanocomposites, Graphene-Based Polymer Nanocomposites in Electronics, Springer Series on Polymer and Composite Materials. In: KK Sadasivuni, Springer International Publishing, Switzerland, 25-47.
- (2015) Final Phase I Report for contract NNX15CG25P and its supplement.
Author Details
Xueying Yang1, Jijue Li1, Xinkun Song1, Yingzhao He1, Xiaoxiao Du1, Yongjin Zou1,2* and Haitao Zhang1,2
1Guangxi Key Laboratory of Information Materials, Guilin University of Electronic Technology, PR China
2Guangxi Collaborative Innovation Center of Structure and Property for New Energy Materials, PR China
Corresponding author
Yongjin Zou, Guangxi Key Laboratory of Information Materials, Guilin University of Electronic Technology, Guilin, 541004, PR China, E-mail: [email protected]
Accepted: November 11, 2017 | Published Online: November 13, 2017
Citation: Yang X, Li J, Song X, He Y, Du X, et al. (2017) Facile Synthesis of Polyaniline-Prussian Blue Composite for High- Performance Supercapacitors. Int J Nanoparticles Nanotech 3:011.
Copyright: © 2017 Yang X, et al. This is an open-access article distributed under the terms of the Creative Commons Attribution License, which permits unrestricted use, distribution, and reproduction in any medium, provided the original author and source are credited.
Abstract
Polyaniline (PANI) and Prussian Blue (PB) composite was firstly fabricated by in-situ chemical polymerization. The morphology and composition of the PANI-PB nanocomposite was characterized Scanning Electron Microscopy (SEM) and Fourier-Transform Infrared Spectroscopy (FTIR). The results indicated that the PANI was well coated on the PB nanoparticles. The PANI-PB composite exhibits excellent electrochemical performance with a specific capacitance of 218.4 F g-1 at a current density of 5 A g-1. Furthermore, PANI-PB nanocomposite shows good stability, which can retain a specific capacitance of 94.2% after 2000 cycles. The good electrochemical performance of the PANI-PB electrode demonstrates that it is suitable for practical applications.
Keywords
Polyaniline, Prussian blue, Supercapacitor, Composite
Introduction
In recent years, energy-storage device research has attached extensive attentions, encouraged by the urgent need of green energy sources. As an development of classic capacitors, supercapacitors, are one of the most promising technologies for future energy storage applications, from memory portable device to electric cars [1-4]. As a matter of fact, the supercapacitor is considered a promising alternative to batteries in the future. According the charge storage mechanisms, supercapacitors can be divided in two categories, including Electric Double-Layer Capacitors (EDLCs) and pseudocapacitors. Specifically, an EDLC stores energy through a non-Faradaic process. In this way, charges are stored electro statically at the electrodes' interfaces. While pseudocapacitors can storage the energy in a Faradaic process, the charge storage is accomplished by electron transfer through changes in the oxidation state of the electrode material. A Faradaic redox reaction depends on reactant ions, and the reaction site density can provide an energy density that is 10-100 times that of a non-Faradaic process [4,5]. Therefore, supercapacitors based on Faradaic processes have generated considerable interest among researchers in recent years. Conducting polymers, transition metal compounds are commonly used materials for pseudocapacitors [3,6-10].
Prussian Blue (PB) is a mixed-valence iron cyanide complex with a repeating unit of potassium or sodium ferrous ferricyanide hexahydrate, which has been considered as the most promising electrode materials for pseudocapacitors because of their excellent electrochemical behavior, good reversibility, and low cost [3,4]. However, PB is unstable in alkali solution. Though it has high specific capacitance, the poor cycling properties limits its applications in supercapacitors. On the other hand, conducting polymer has good stability and high capacitance. Therefore, we hypothesis that the conducting polymer protected PB composite may potentially improve the stability of PB.
In this study, a simple chemical method was explored to prepare PANI-PB composite. The PANI-PB system exhibited a fine synergy between the PB and PANI, with the obtained nanocomposite demonstrating a high specific capacitance and good stability.
Materials and Methods
Preparation of PANI-PB composites
All the chemicals are analytical grade and used as received, except for aniline, which was distilled at reduced pressure before use. PANI-PB was prepared by in-situ chemical polymerization. Typically, 50 mL of a mixed solution of 0.02 mol L-1 FeCl3, 0.02 mol L-1 K3Fe[CN]6, and 0.1 mol L-1 HCl was added drop wise to 50 mL of a 0.1 mol L-1 aniline solution under vigorous stirring. The suspension was stirred for 12 h. The resulting solid was filtered and rinsed thoroughly with water and then ethanol. Then the sample was left to dry in a vacuum oven at 60 ℃ for 24 h. For comparison, PB and PANI were prepared with the identical conditions. Fourier-Transform Infrared (FTIR) spectra of all the samples were recorded using a Nicolet 6700 spectrometer (USA). Scanning Electron Microscopy (SEM; Quanta 200, FEI, USA) and transmission electron microscopy (TEM; JEM2010, JEOL, Japan) were used to characterize the morphology and surface composition of the samples.
Electrochemical tests
The working electrodes for the electrochemical capacitors were prepared by mixing 50 mg of the synthesized samples with 30 mg of carbon black and 20 mg of polyvinylidene fluoride as the binder to form slurries. Next, 100 μL of ethanol was added to the mixtures. The slurries were coated onto nickel foam current collectors using a blade. The active material of the electrode was 50 mg. The content of PB was roughly calculated to be 64% in the composite from the starting materials. The resulting electrodes were dried at 60 ℃ for 6 h. Conventional three electrode system was set up to study the electrochemical performance of the PANI-PB electrode. A platinum electrode and a saturated calomel electrode were used as the counter and reference electrodes, respectively. The electrolyte was a 6 M KCl solution. Cyclic Voltammetry (CV) and Galvanostatic Charge-Discharge (GCD) measurements were performed using an IM6e electrochemical workstation (Zahner-Elektrik, Kronach, Germany). The specific capacitances of the electrodes were calculated using established equations [2]. All the experiments were performed at room temperature.
Results and Discussion
Characterization of the catalysts
Figure 1 shows the SEM and TEM images of the prepared PANI-PB samples. The SEM image in Figure 1a shows that PB was aggregated nanoparticles. The morphology of PANI was composed uniform nanosphere. The diameter of the PANI particles is about 50 nm (Figure 2b). After doped with PB during the polymerization process, the separated PB nanoparticles were coated the PANI film (Figure 2c). The TEM image of PANI-PB further confirmed the PB nanoparticles were coated by PANI film (Figure 2d). Generally, the preparation of PB nanoparticles doped composite materials are complicated, which restrained their application in electrochemial device. In this work, the PANI-PB nanocomposite was synthesized in a facile way. FeCl3 was enlisted as an effective oxidant for polyaniline, which offers a good conductivity and high synthesis rate. At the same time, the PB nanopartilce were formed.
To investigate the chemical composition of the PANI-PB nanocomposite, the FTIR spectroscopy was recorded. Figure 2 shows the FTIR spectra of PB, PANI and the PANI-PB nanocomposite. It can be seen that pure PB shows a strong peak at 2090 cm-1, which is ascribed to the stretching absorption band of the CN group in the Fe2+-CN-Fe3+. This band can also be observed in the PANI-PB spectrum. In addition, the absorption bands near 3415 and 1610 cm-1, attributable to the O-H stretching and H-O-H bending modes, respectively, indicate the presence of interstitial water in the samples. For the spectrum of PANI, the peaks at 1483 cm-1 can be assigned to the C = C stretching vibration of quinoid [5]. This peak of PANI can also be found in the composite of PANI-PB. These results confirm that the PANI-PB composite was successfully synthesized.
To access the electrochemical performance of the composite, Cyclic Voltammetries (CVs) were performed in 6.0 M KNO3 solution. Figure 3 shows the CV curves of the PB, and PANI-PB at the scan rate of 10 mV/s. It can be seen that the PANI-PB electrode displayed obvious symmetric redox peaks, indicating a good reversibility of the electrochemical reaction. A pair of redox peakswas observed at around 0.2 V, which is assigned to the redox process of the Fe2+/Fe3+ couple coordinated via nitrogen atoms from C-N group [11]. Besides the redox peak from the PB, the CVof PANI-PB electrode increases significantly, indicating that synergistic effect bewteen the PB and PANI. The high CV current indicates the high conductivity and low internal resistance for PANI-PB composite as electrode materials.
Figure 4 compares the GCD curves for the different electrodes at a current density of 5 A g-1. The specific capacitances were calculated as 35.6, 85.2, and 218.4 F g-1 for the PB, PANI, and PANI-PB electrodes, respectively. The symmetric GCD curves of the PANI electrode are linear with constant slopes for the total range of potentials. For the PB and PANI-PB electrodes, two obviously different sections appear in the discharge curves, including a fast potential drop followed by a slow potential decay; the former is attributed to the internal resistance of the electrode and the latter represents the capacitive features of the pseudocapacitive electrode [7,9,12]. The nonlinear curve shapes of the PB and PANI-PB electrodes are typical pseudocapacitance behavior. Compared with the PB electrode, the PANI-PB electrode has lower internal resistance and higher pseudocapacitance. The specific capacitance of the PANI-PB is about 6 times larger than that of PB electrode.
Figure 5 shows the GCD curves of PANI-PB composite at different current densities of 5, 10, 15, 20, and 25 A g-1. The triangular shapes of the GCD curves indicate good reversibility and capacitive performance during charging/discharging. The PANI-PB composite preserves 56.7% of its initial specific capacitance value as the current density is increased from 5 to 25 A g-1, indicating its good high-rate capability.
Cycling stability is an important factor for the supercapacitors. To investigate the stability of the PANI-PB electrode, the PANI-PB hybrid electrode was cycled at 5 A g-1 over 2000 cycles. Figure 6 shows the plots of the resulting normalized capacitance retention of the electrode as a function of the cycle number. The PANI-PB electrode demonstrated a good long-term cycling stability, retaining 94.2% of its initial capacitance after 2000 cycles, which indicates its excellent cycling stability and high degree of reversibility in repetitive charge/discharge cycling. The results suggest that PANI may possibly stabilize the PB particles by keeping them mechanically surface-bound. Such strong structural integrity would suppress the dissolution and agglomeration of the active materials during the cycling tests.
Conclusions
PANI-PB nanocomposites were successfully prepared as supercapacitor electrode materials and subsequently demonstrated excellent supercapacitor performance. The resultant supercapacitor exhibits good pseudocapacitance behaviors. It demonstrates excellent cyclic stability, with 94.2% capacitance retention for up to 2000 cycles, and provides a maximum specific capacitance of 218.4 F g-1 at a current density of 5 A g-1. Therefore, we believe that this hybrid supercapacitor based on the PANI-PB nanocomposite holds the potential to be applied in the areas such as supercapacitor, battery, catalysis and capacitive mixing technique.
Acknowledgements
This work was supported by the Guangxi undergraduate innovation and entrepreneurship training program (201610595187), the GNSF (2016GXNSFFA380012, 2014GXNSFAA118318, 2014GXNSFBA118240, and 2015GXNSFAA139282).