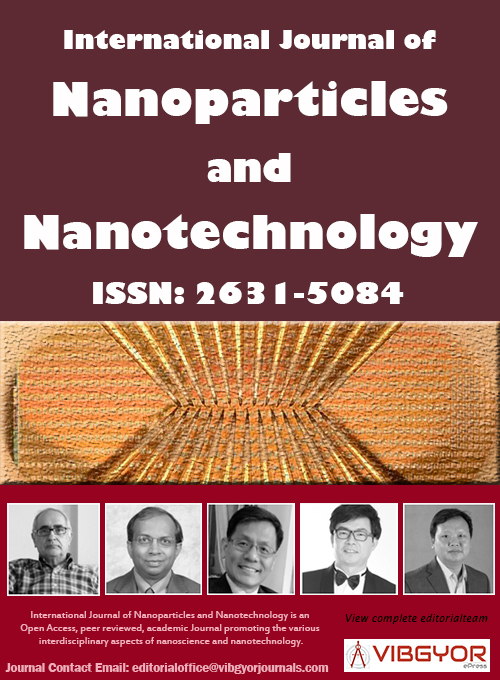
International Journal of Nanoparticles and Nanotechnology
(ISSN: 2631-5084)
Volume 8, Issue 1
Research Article
DOI: 10.35840/2631-5084/5541
Effect of Asymmetric Terminal Group on the Transport Properties of Molecule Junctions: A First-Principles Study
Jing-Xin Yu*, Zhi-Yu Hou, Wan-Jie Ding, Xiu-Ying Liu and Xiao-Dong Li
Table of Content
Figures
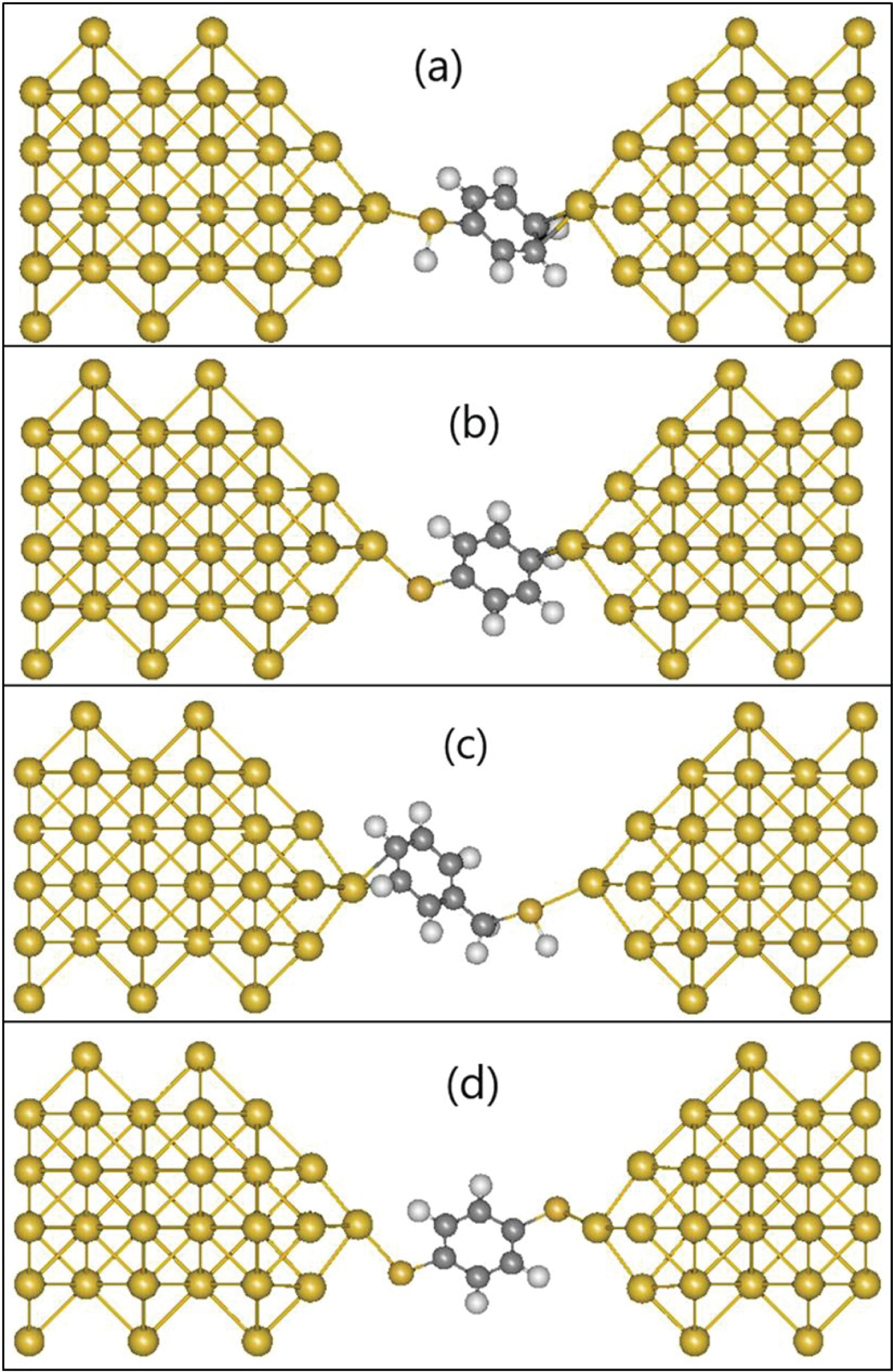
Figure 1: Device geometries: (a) Thiophenol...
Device geometries: (a) Thiophenol (TP); (b) C6H5S; (c) Benzyl mercaptan (BMP); (d) C6H4S2 attached to gold (100) electrodes.
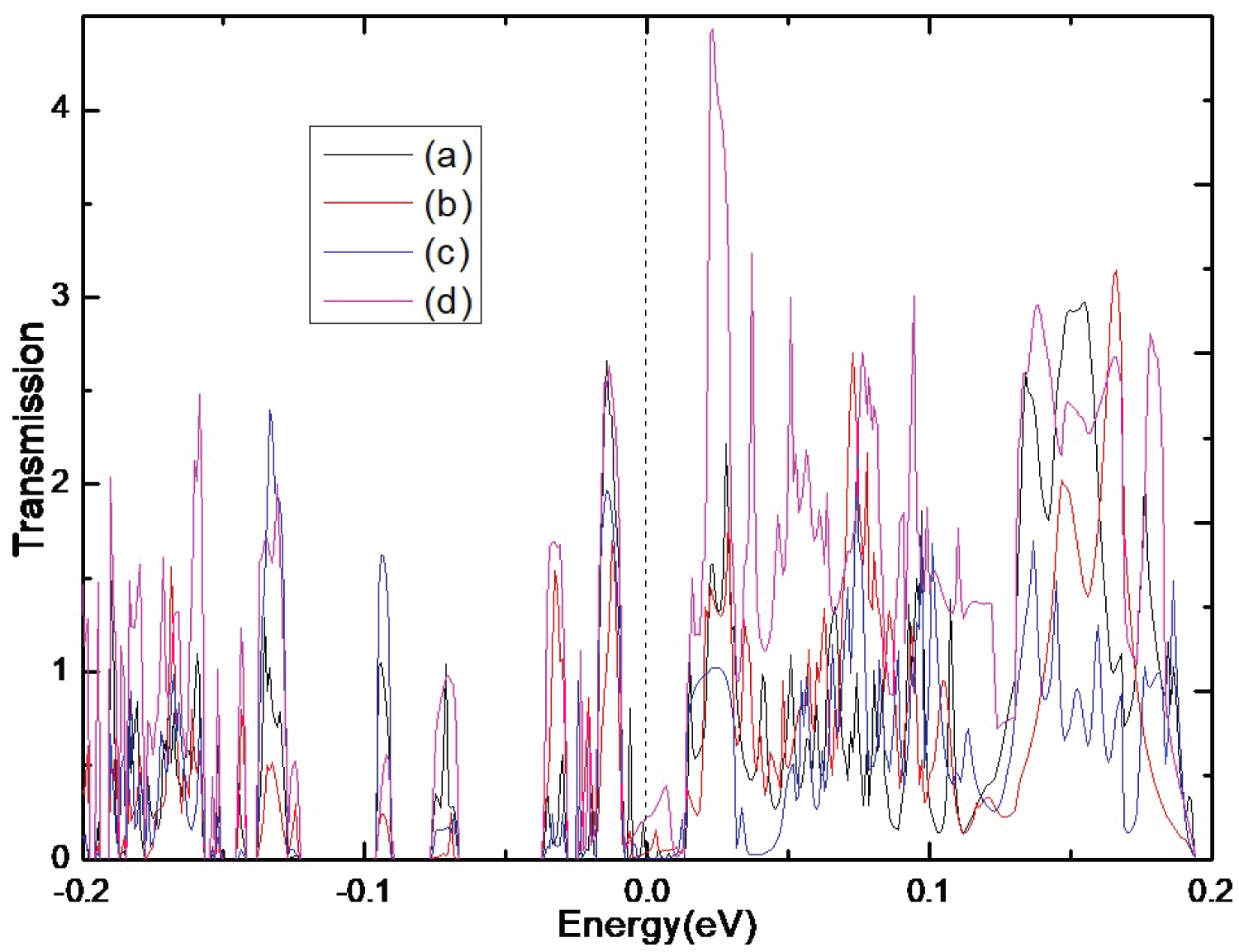
Figure 2: The transmission coefficients....
The transmission coefficients at zero biasof all the considered geometries.
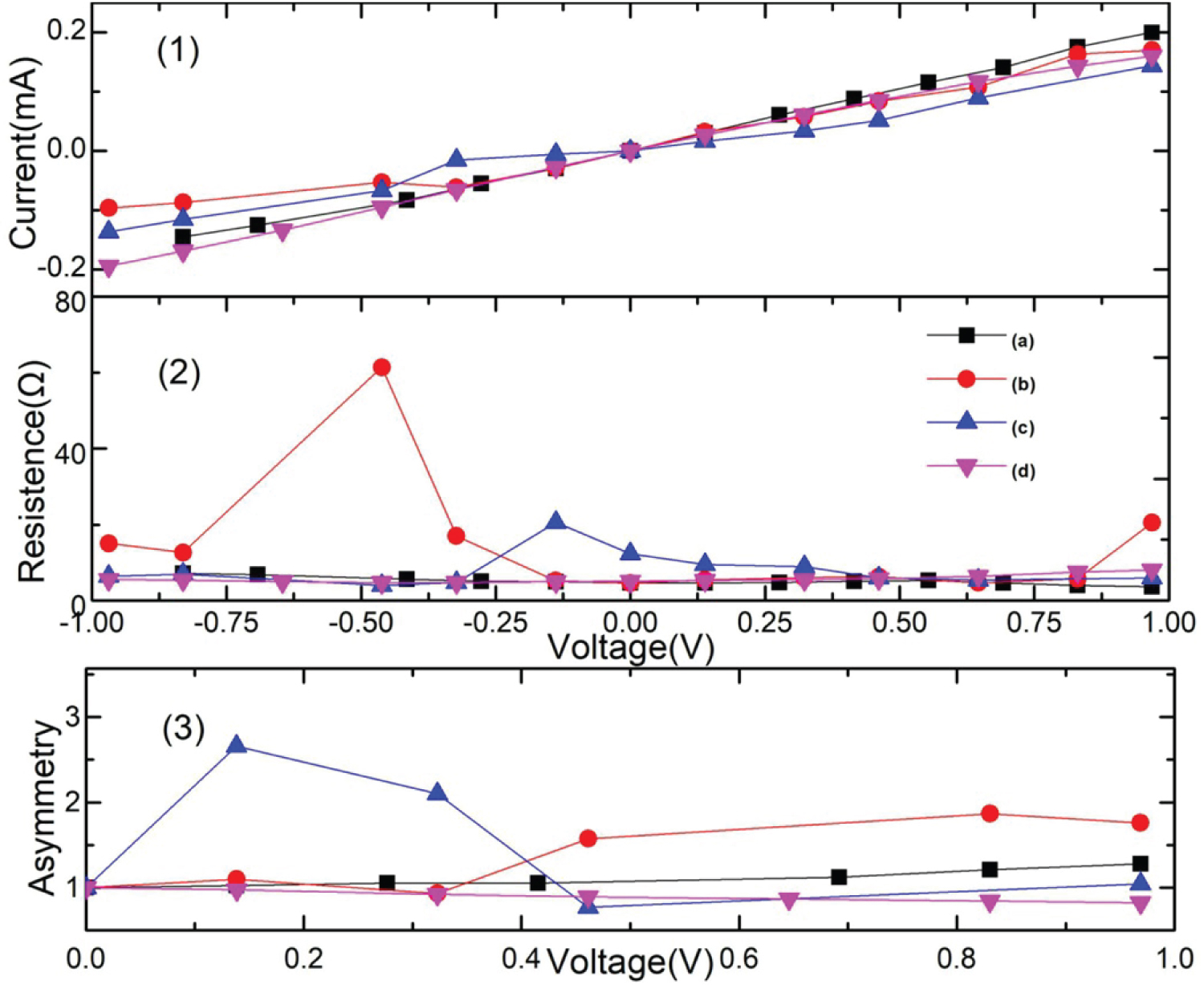
Figure 3: (1) I-V characteristics,....
(1) I-V characteristics, (2) differential resistance and (3) asymmetry of all the considered geometries.
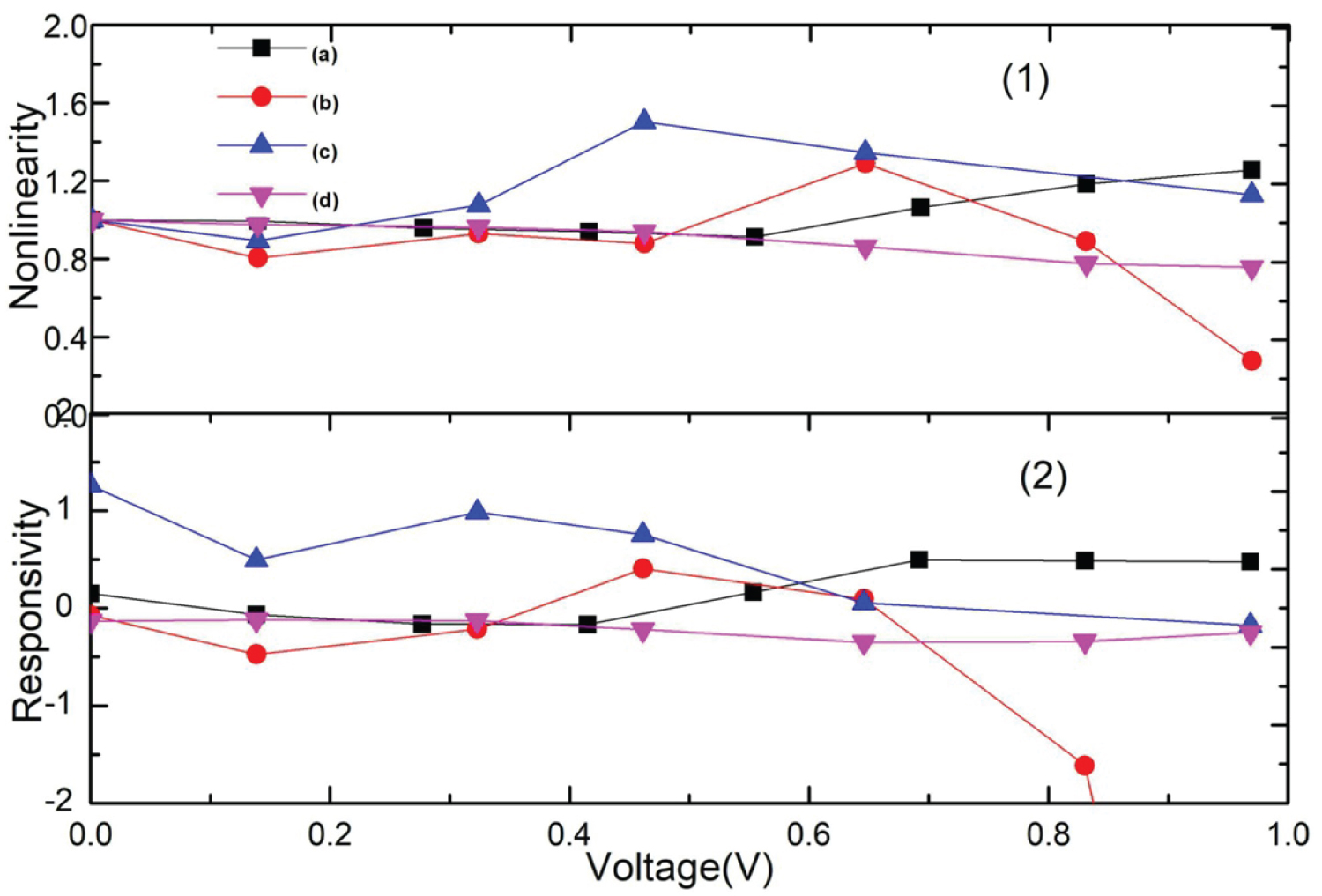
Figure 4: (1) Nonlinearity and....
(1) Nonlinearity and (1) responsivity of the considered molculars.
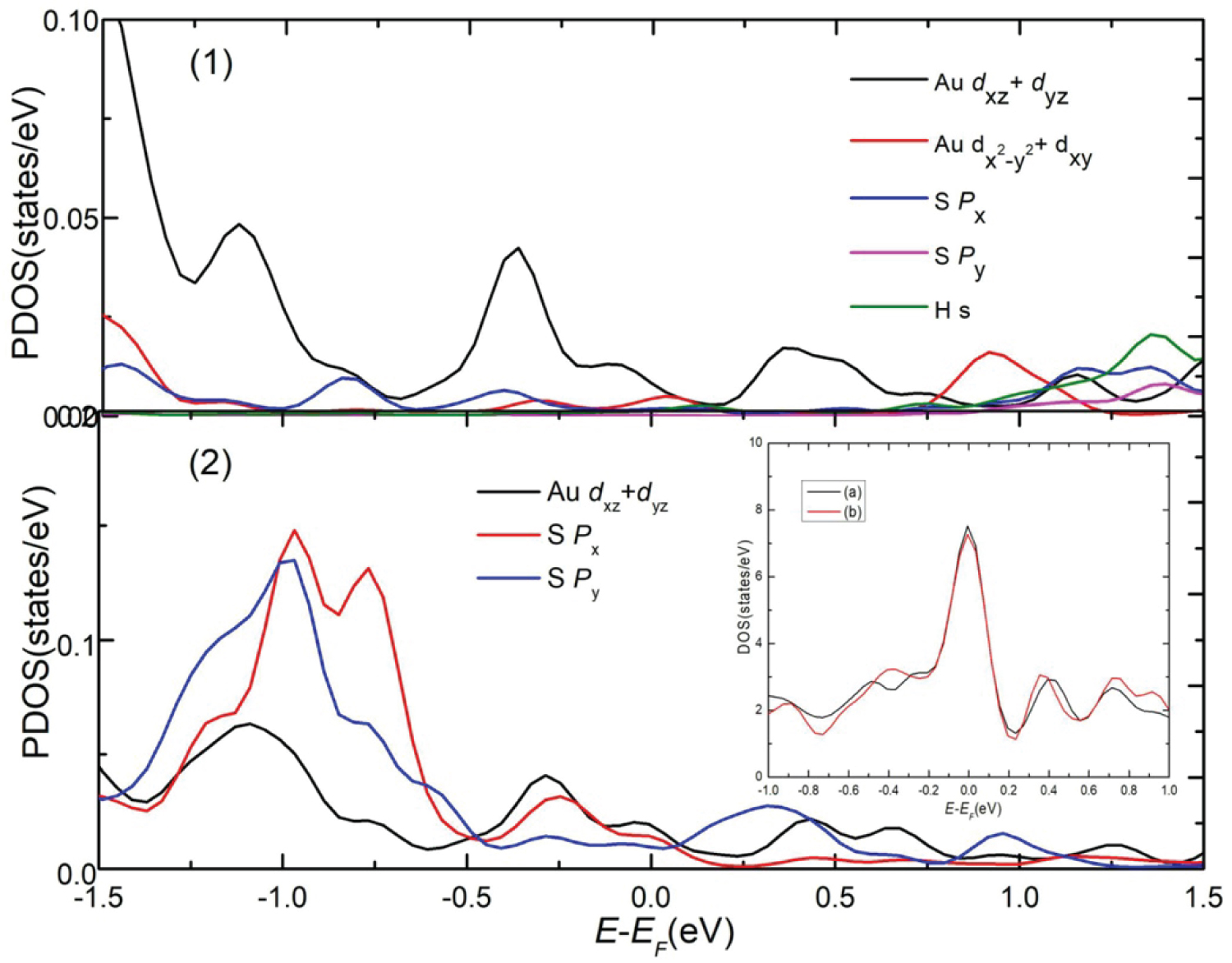
Figure 5: (1) ThePDOS of the considered....
(1) ThePDOS of the considered (a) TP molcular and (2) the PDOS of the (b) C6H5S molcular. Embedded graphics is the DOS of the considered molculars (a) and (b), respectively.
References
- Batra A, Darancet P, Chen QS, Meisner JS, Widawsky JS, et al. (2013) Tuning rectification in single-molecular diodes. Nano Lett 13: 6233-6237.
- Bao DL, Liu R , Leng JC , Zuo X, Jiao Y, et al. (2014) Theoretical study on mechanical and electron-transport properties of conjugated molecular junctions with carboxylic or methyl sulfide links. Physics Letters A 378: 1290-1295.
- Fan SW, Wang RG (2018) Effect of electrode position and cross section size on transport properties of molecular devices. Acta Phys Sin 67: 213101.
- Rodrigo D, Limaj O, Janner D, Etezadi D, García de Abajo FJ, et al. (2015) Mid-infrared plasmonic biosensing with grapheme. Science 349: 165-168.
- Galperin M, Ratner M, Nitzan A, Troisi A (2008) Nuclear coupling and polarization in molecular transport junctions: Beyond tunneling to function. Science 319: 1056-1060.
- Chen X, Chen YH, Qin J, Zhao D, Ding B, et al. (2017) Mode modification of plasmonic gap resonances induced by strong coupling with molecular excitons. Nano Letters 17: 3246-3251.
- Ni WH, Ambjornsson T, Apell SP, Chen HJ, Wang JF (2010) Observing plasmonic-molecular resonance coupling on single gold nanorods. Nano Lett 10: 77-84.
- Ren J, Tian K, Jia L, Han X, Zhao M (2016) Rapid covalent immobilization of proteins by phenol-based photochemical cross-linking. Bioconjug Chem 2: 2266-2270.
- Gopinath A, Rothemund PWK (2014) Optimized assembly and covalent coupling of single-molecule DNA origami nanoarrays. ACS Nano 8: 12030-12040.
- Reddy P, Jang SY, Segalman RA, Majumdar A (2007) Thermoelectricity in molecular junctions. Science 315: 1568-1571.
- Parker SM, Smeu M, Franco I, Ratner MA, Seideman T (2014) Molecular junctions: Can pulling influence optical controllability? Nano Lett 14: 4587-4591.
- Benz F, Tserkezis C, Herrmann LO, de Nijs B, Sanders A, et al. (2015) Nanooptics of molecular-shunted plasmonic nanojunctions. Nano Lett 15: 669-674.
- Hu PC, Li X, Li B, Han XF, Zhang FR, et al. (2018) Molecular coupling between organic molecules and metal. J Phys Chem Lett 9: 5167-5172.
- Van DC, Ratner MA (2015) Molecular rectifiers: A new design based on asymmetric anchoring moieties. Nano Lett 15: 1577-1584.
- Berdiyorov GR, Hamoudi H (2021) Effect of anchoring groups on the electronic transportproperties of biphenyl and phenyl-pyridine molecules. J Mater Res Technol 12: 193-201.
- Kanthasamy K, Pfnu¨r H (2015) Conductance through single biphenyl molecules: symmetric and asymmetric coupling to electrodes. Beilstein J Nanotechnol 6: 1690-1697.
- Jamali MF, Soleimani HR, Tagani MB (2019) The maximum rectification ratio of pyrene-based molecular devices: A systematic study. J Comput Electron 18: 453-464.
- Koga J, Tsuji Y, Yoshizawa K (2012) Orbital control of single-molecule conductance perturbed by p-accepting anchor groups: Cyanide and isocyanide. J Phys Chem C 116: 20607-20616.
- Lan TN (2014) Electronic transport properties of molecular junctions based on the direct binding of aromatic ring to electrodes. Chem Phys 428: 53-58.
- Li MJ, Xu H, Chen KQ, Long MQ (2012) Electronic transport properties in benzene-based heterostructure: Effects of anchoring groups. Phys Lett A 376: 1692-1697.
- Qiu S, Miao YY, Zhang GP, Ren JF, Wang CK, et al. (2019) Enhancement of magnetoresistance and current spin polarization in single-molecule junctions by manipulating the hybrid interface states via anchoring groups. J Magn Magn Mater 479: 247-253.
- Lim JK, Kwon O, Joo SW (2008) Interfacial structure of 1,3-Benzenedithiol and 1,3-Benzenedimethanethiol on silver surfaces: Surface-enhanced raman scattering study and theoretical calculations. J Phys Chem C 112: 6816-6821.
- Rocha AR, Garcia-Suarez VM, Bailey S, Lambert C, Ferrer J, et al. (2006) Spin and molecular electronics in atomically generated orbital landscapes. Phys Rev B 73: 085414.
- Rungger I, Sanvito S (2008) Algorithm for the construction of self-energies for electronic transport calculations based on singularity elimination and singular value decomposition. Phys Rev B 78: 035407.
- Kadanoff LP, Baym G (1962) Quantum Statistical Mechanics. Benjamin-Cummings, New York.
- Datta S (1995) Electronic transport in Mesoscopic system. Cambridge University Press, Cambridge.
- Kohn W, Sham LJ (1965) Self-consistent equations including exchange and correlation effects. Phys Rev 140: 1133-1138.
- Soler JM, Artacho E, Gale JD, Garcia A, Junquera J, et al. (2002) The SIESTA method fou ab initio order-N materials simulation. J Phys Condens Matter 14: 2745-2779.
- Perdew JP (1986) Density functional approximation for th correlation energy of the homogeneous electron gas. Phys Rev B Condens Matter 33: 8822-8824.
- Troullier N, Martins JL (1991) Efficient pseudopotentials for plane-wave calculations. Phys Rev B Condens Matter 43: 1993-2006.
- Frisenda R, Tarkuc S, Galan E, Perrin ML, Eelkema R, et al. (2015) Electrical properties and mechanical stability of anchoring groups for single-molecule electronics. Beilstein J Nanotechnol 6: 1558-1567.
Author Details
Jing-Xin Yu*, Zhi-Yu Hou, Wan-Jie Ding, Xiu-Ying Liu and Xiao-Dong Li
College of Science, Henan University of Technology, Zhengzhou, China
Corresponding author
Jing-Xin Yu, College of Science, Henan University of Technology, Zhengzhou 450001, China.
Accepted: February 13, 2023 | Published Online: February 15, 2023
Citation: Jing-Xin Y, Zhi-Yu H, Wan-Jie D, Xiu-Ying L, Xiao-Dong L (2023) Effect of Asymmetric Terminal Group on the Transport Properties of Molecule Junctions: A First-Principles Study. Int J Nanoparticles Nanotech 8:041.
Copyright: © 2023 Jing-Xin Y, et al. This is an open-access article distributed under the terms of the Creative Commons Attribution License, which permits unrestricted use, distribution, and reproduction in any medium, provided the original author and source are credited.
Abstract
Single-molecule quantum transport calculations have been investigated using non-equilibrium Green's function method combined with the density functional theory (DEF-NEGF) for phenyl molecules symmetrical or asymmetrically anchored to Au(100) electrodes via different terminal groups. Among the considered 4 structures, the electronic coupling of C6H5S is slightly stronger than thiophenol. Because the -S as terminal group provides more free electrons than -SH, this results in significant current rectification, but the stability of responsivity is poorer than thiophenol molecule. Thiophenol molecules show good diode parameters in terms of asymmetry, nonlinearity and responsiveness above 0.5V the applied voltage. Although there is only one H atom differences, the thiophenol and C6H5S molecular show different property. The structure and diode characteristics of Thiophenol molecule are conducive to become interface modification materials and improve the photoelectric performance of devices.
PACS numbers: 71.15.Mb;61.46.-w; 73.40.c
Keywords
Molecular junction, Electron transport, Non-equilibrium Green's function
Introduction
In recent decades, to investigate the intrinsic quantum phenomena, the charge transport characteristics of single molecule devices have been widely studied. As we all know, different terminal groups of molecules will lead to different interactions between molecular junctions and electrodes for molecular junction systems [1-3]. Molecular coupling at the interface plays an important role in determining the performance of molecular electronics, such as the immobilization of molecular devices [4-9]. Molecules with phenyl groups have been widely employed in molecular electronics because of their high electron transfer properties [10-12]. Thiol(SH) group is considered as a potential anchoring group, which it can construct mechanically stable single molecule junctions in molecular junctions. The bridged methylene group is also commonly used to regulate the molecular coupling between the phenyl molecules and surface free electrons [13]. Due to the surface catalysis, the H atom in -SH is easy to be dissociated. Therefore, for the molecular system with -SH as the terminal group, the S atom forms a covalent bond with the gold electrode. In order to understand the difference of terminal groups on the electron transport properties, we have designed different molecular systems with gold as electrode, benzene as molecular skeleton, -S, -SH as terminal groups and -CH2 bridging respectively.
In addition, the asymmetric anchoring between the molecular junction and electrode can change the transport properties, such as it can be used to create molecular rectifiers [14]. Many novel phenomena also have obtained, current rectification, negative differential resistance, etc [15-21]. Obviously, it is necessary to study the asymmetric anchoring and the coupling between molecular containing phenyl groups and free electrons on the metal surfaces.
We present the combined electrode-molecular system together with seven and six Au(100) atomic layers respectively to the left- and right-hand surface plane, each layer containing 3 × 3 atoms in the xy plane with periodic boundary conditions. Figure 1 shows the considered four molecular structures.
a) Thiophenol (TP), which shows good diode parameters in terms of asymmetry, nonlinearity and responsiveness.
b) C6H5S. Compared with TP molecule, it is observed the larger current and significant current rectification.
c) Benzyl mercaptan (BMP). The methylene group improves mechanical stability of the junctions [22].
d) C6H4S2. We use symmetric structure as a comparison of the transport properties of the other three asymmetric molecular junction system. We have analyzed the effect of H bond in terminal groups on the transport properties, and analyzed the influence of different terminal groups on the diode parameters of Thiophenol molecular.
Model and Method
The electronic transport properties of molecular junctions have been calculated with the SMEAGOL software [23-27]. We first have performed primary geometric optimization for the molecular of the central region, the molecules along with nearest two layers Au are relaxed and four and five outer Au layers respectively to the left- and right-hand surface plane are frozen in their bulk position. Then the transport properties of molecular junctions, including the electron transmission coefficients of zero-bias, current- voltage (I-V) curves, the project density of states (PDOS), are calculated on the basis of optimization results [28]. We also calculated the resistance, nonlinearity, responsivity, and asymmetry of all the considered systems:
(differential resistance)
(nonlinearity)
(responsivity)
(asymmetry) [4].
Our calculations are carried out using DFT-NEGF with the Perdew-Zunger form [29] of the local-density approximation (LDA) to the exchange-correlation functional. Troullier-Martins pseudo potentials [30] are used for Au (5d106s1). A single-zeta polarization (SZP) is used for Au atoms, whereas a double-zeta polarization (DZP) basis set is adopted for the orbitals of S, C and H atoms.
Results and Discussion
The ground state energy of the electrode-molecular system is calculated. By comparing the (a) TP and (b) C6H5S molecules, we find that their optimized structures are similar. Due to the presence of H in sulfhydryl group, the Au-S bond (about 2.59 Å) in (a) TP molecule is longer than the (b) C6H5S molecule (about 2.39 Å). In (a) TP molecule, the gold electrode at the other end is bonded with two C atoms in benzene ring. However, the gold electrode is bonded to a C atom in the benzene ring in (b) C6H5S molecule. The (a) TP molecule is more stable than (b) C6H5S molecule. In (c) BMP molecule, the Au-S bond about 2.58 Å and the gold electrode at the other end is bonded with two C atoms in benzene ring. This is similar to (a) TP molecule.
In Figure 2, we display the transmission spectra of all the considered systems at zero bias. The conductance at zero bias is 0.01 G0(G0 = 2e2/h), 0.03 G0,0.001 G0 and 0.22 G0 for the (a) TP, (b) C6H5S, (c) BMP and (d) C6H4S2 molecules, respectively. Because of the weak resonance, the highest occupied molecular orbital (HOMO) transmission peaks of all the molecular junctions is small near the Fermi level (EF), but the effect of terminal groups on the conductivity of the molecular junctions is clearly observed. As expected, (d) shows a higher peak, so it corresponds to a large transmission probability, the essential reason of the large conductance due to strong sulfur-gold bonding with both electrodes. In the case of molecule structure (b), -S as terminal groups bonding with left electrode provides one more free electron than -SH, so the electronic coupling is slightly stronger than the structure (a). In the case of molecule structure (c), the molecular coupling is weakest because of the existence of bridged methylene group. In fact, the molecule structure (c) has the smallest transmission among all the considered systems. The above result is also agreement with the previous experimental results [14].
Figure 3 presents the calculated I-V curves, differential resistance and asymmetry of all models. As depicted in Figure 3(1), because of the strong S-Au bonds and better electronic level alignment [31], the molecule structure (d) have been observed the larger current in the whole bias voltage range (filled-magenta triangles in Figure 3(1)). Interestingly, the current in structure (d) has no obvious advantage as compared to the structure (b) despite only covalent S-Au bonding to left electrode. For the structure (c) (filled-blue triangles in Figure 3(1) and Figure 3(2)), the -CH2 bridging may cause weak interfacial electronic coupling, it displays the smallest current but the largest differential resistance. In Figure 3(2), the differential resistance is small except for the structure (c), indicating that they belongs to the molecular resistance. The structure (a) and (b) shows obvious asymmetric characteristics because of the structural asymmetry, the current is larger for the positive bias and the current is smaller for the negative voltages (filled-black squares and filled-red circles in Figure 3(1)). Figure 3(3) presents the asymmetries of all models, the larger current rectification is obtained for structure (a) and (b), the result is also good agreement with the Figure 3(1). Although there is only the difference of one atom, the asymmetry of the structure (b) increases more than the structure (a) above 0.5 V the applied voltage. The structure (a) shows the better stability in a symmetry for positive voltage biasing. So the hydrogen bond coordination in molecular junction maybe plays an important role in electronic coupling.
Figure 4(1,2) show the nonlinearity and responsivity of all the models. For the structure (b), it is observed clear current rectification because of the -S as terminal group, and the maximum rectification ratio is 1.8. But its stability of responsivity is poorer than the structure (a). The structure (c) and (a) shows better nonlinearity and responsivity in the whole bias voltage range (filled-blue triangles). However, the structure (c) has no practical value due to the small current. In summary, the structure (a) shows better diode parameters in all the considered molecular junctions.
The electronic transport mechanism also can be attributed to the PDOS of the molecular region (Figure 5). Here, we present the results only for (a) TP and (b) C6H5S molecular because of their better conductance and diode characteristics. Embedded graphics is the DOS of the considered molecular (a) and (b), there is no obvious difference and the trend is consistent with the transport calculation results. For the molecular junction (a) (see Figure 5(1)), the effective electron transport channel is mainly originate from the Au dxz and dyz orbitals. For the molecular junction molecule (b), the conductance is larger than (a). The effective electron transport channel is mainly formed not only by the Au dxz and dyz orbitals but also by the S Px and Py orbitals, mainly because there are more free electrons -S as terminal groups. This also explains that the current is larger for the positive bias but the current is smaller for the negative voltages (i.e., larger rectification ratio) (see Figure 3(3)).With the application of bias voltage, the S atom Px and Py orbitals plays a decisive role in the difference asymmetry, nonlinearity and responsivity of the molecular (a) and (b).
Conclusions
In the present work, we have examined the effect of different terminal groups on the electronic transport properties using DEF-NEGF for phenyl molecules symmetrical or asymmetrically anchored to Au(100) electrodes. The larger current, asymmetry, nonlinearity and responsivity are obtained for thiophenol molecule above 0.5V the applied voltage. The -S as terminal group of phenyl molecule also is observed clear current rectification, but its stability of responsivity is poorer than thiophenol molecule. Detailed analysis of PDOS shows that the obtained current rectification formed by the effective electron transport channel of Au and S. Although there is only one H atom differences, the thiophenol and C6H5S molecular show different property. The structure and diode characteristics of thiophenol molecule are conducive to become interface modification materials and improve the photoelectric performance of devices. The results have practical importance for understand electron transport behavior and construct the molecular electronics devices.
Acknowledgments
We thank the support plan for key teachers of Henan University of Technology. We also thank Dr. Sanvito for his providing us the SMEAGOL code.