Polymers and TiO2 Based Nanocharges for Bio-Based Composites with Improved Flame Retardancy and Thermal Degradation
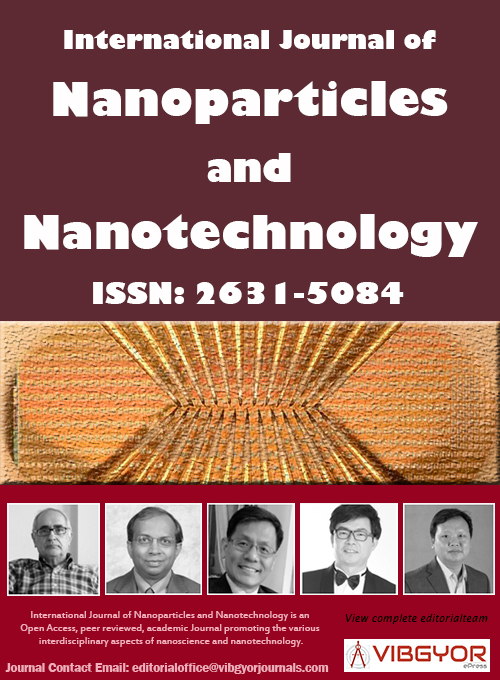
International Journal of Nanoparticles and Nanotechnology
(ISSN: 2631-5084)
Volume 6, Issue 1
Original Article
DOI: 10.35840/2631-5084/5537
Polymers and TiO2 Based Nanocharges for Bio-Based Composites with Improved Flame Retardancy and Thermal Degradation
Nabil Bouazizi*, Ahmed Abed, Stéphane Giraud, Ahmida El Achari, Christine Campagne, Julien Vieillerd and Franck Le Derf
Table of Content
Figures

Figure 1: Optical microscopy of CO and: a) CO-PVDF-TiO2-CT....
Optical microscopy of CO and: a) CO-PVDF-TiO2-CT; b) Composite and SEM images of biobased cotton.

Figure 2: Photograph of colored water immersion-height.....
Photograph of colored water immersion-height during 60 min and changes in drop shape on: a) CO; and b) CO-PVDF-TiO2-CT composites.
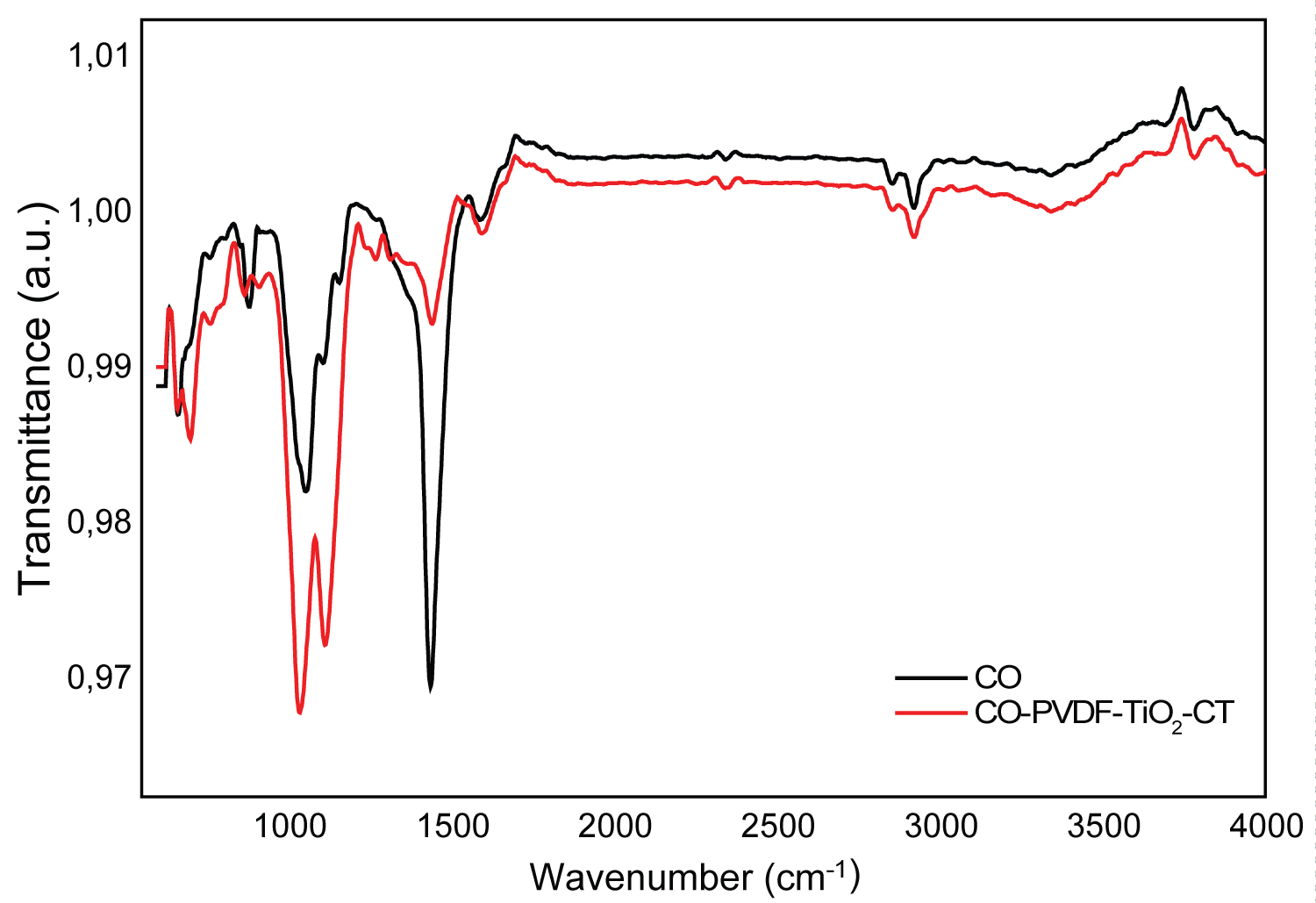
Figure 3: FTIR spectra of CO and CO-PVDF-TiO2-CT....
FTIR spectra of CO and CO-PVDF-TiO2-CT composites.

Figure 5: TGA patterns of original biobased cotton....
TGA patterns of original biobased cotton and: a) CO-PVDF-TiO2-CT composites.

Figure 6: Flame retardancy tests on original....
Flame retardancy tests on original CO: a) Before ignition; b) After ignition, and on the CO-PVDF-TiO2-CT composites; c) Before ignition; d) After ignition.
Schemes

Scheme 1: Postulated ternary system as composite....
Postulated ternary system as composite for flame retardancy application.

Scheme 2: Synthesis and functionalization of.....
Synthesis and functionalization of biobased cotton.
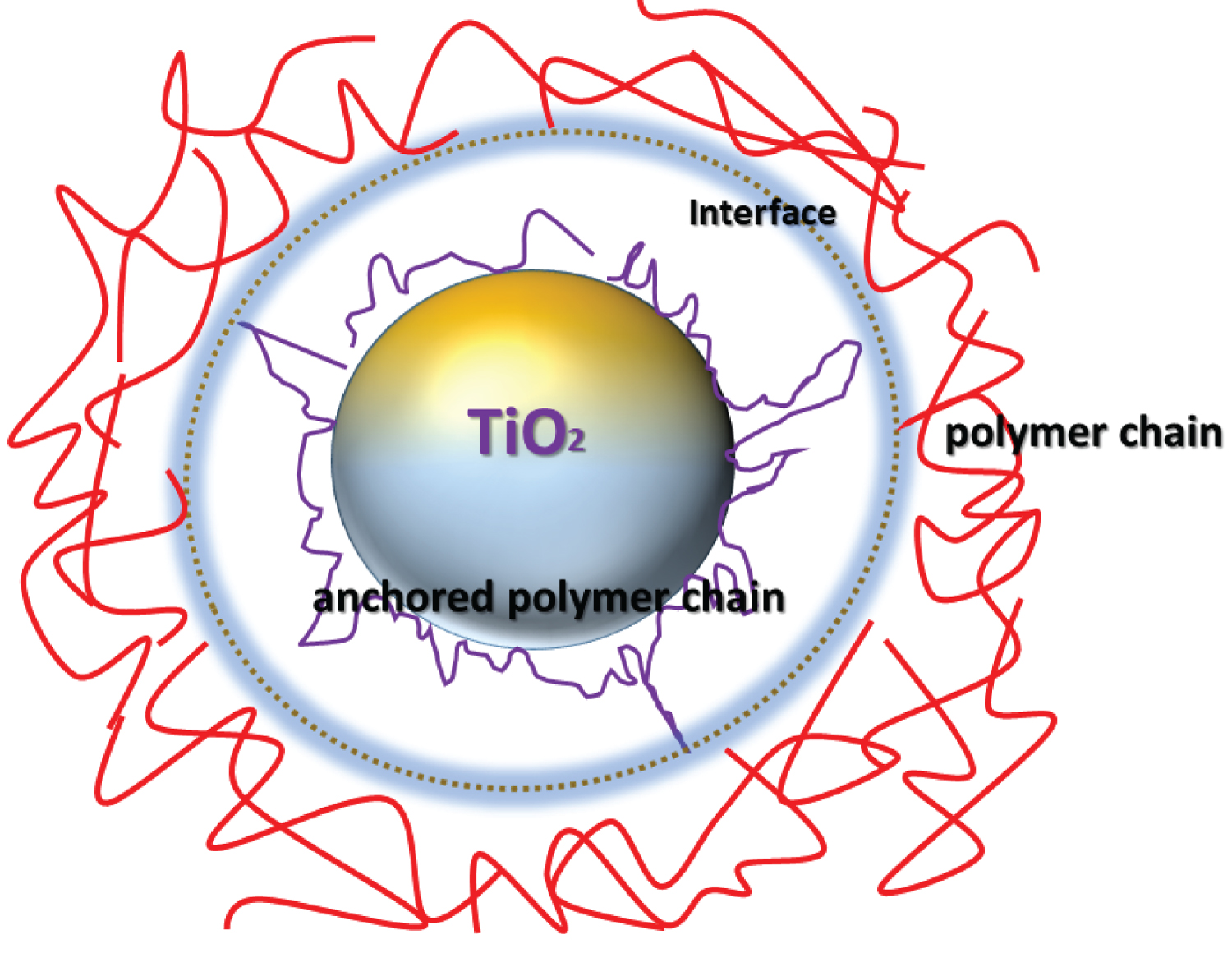
Scheme 3: Proposed adsorption of polymer chains....
Proposed adsorption of polymer chains on the surface of the metal oxide.

Scheme 4: Possible intervention of hydroxyl groups....
Possible intervention of hydroxyl groups present on the surface of TiO2 particles with biobased cotton.
Tables
Table 1: Evolution in time of the contact angle for CF and modified counterparts.
Table 2: TGA data of PET and modified counterparts.
Table 3: Main features in flame retardancy test of CO and its modified counterparts.
References
- L Zhi, SIM Lira, L Zhang, F Daniel, B Vignesh, et al. (2018) Bio-inspired engineering of boron nitride with iron-derived nanocatalyst toward enhanced fire retardancy of epoxy resin. Polymer degradation and stability 157: 119-130.
- L Yun, W Quan-Quan, J Zhi-Ming, CJ Zhang, ZF Li, et al. (2018) Effect of chitosan on the fire retardancy and thermal degradation properties of coated cotton fabrics with sodium phytate and APTES by LBL assembly. Journal of Analytical and Applied Pyrolysis 135: 289-298.
- L Teng, L Shuai, M Tongjun, Y Zhong, L Zhang, et al. (2019) Novel organic-inorganic hybrid polyphosphazene modified manganese hypophosphite shuttles towards the fire retardance and anti-dripping of PET. European Polymer Journal 120: 109270.
- L Lubin, X Yue, H Yintong, M Xu, Z Shi, et al. (2019) An effective mono-component intumescent flame retardant for the enhancement of water resistance and fire safety of thermoplastic polyurethane composites. Polymer Degradation and Stability 167: 146-156.
- K Katarzyna, O Paulina, R Urszula, W Szaniec (2019) Assessment of steel truss fire safety in terms of the system reliability analysis. Archives of Civil and Mechanical Engineering 19: 417-427.
- G Raedet, B Hutaf (2009) Determination of decabromodiphenyl ether in backcoated textile preparation. Journal of hazardous materials 162: 249-253.
- Y Sohailet S Danmei (2019) Propelling textile waste to ascend the ladder of sustainability: EOL study on probing environmental parity in technical textiles. Journal of Cleaner Production 233: 1451-1464.
- A Ahmed, B Nabil, G Stéphane, AE Achari, C Campagne, et al. (2020) Polyester-supported chitosan-poly (vinylidene fluoride)-inorganic-oxide-nanoparticles composites with improved flame retardancy and thermal stability. Chinese Journal of Polymer Science 38: 84-91.
- FR Costa, U Wagenknecht, G Heinrich (2007) LDPE/Mg-Al layered double hydroxide nanocomposite: Thermal and flammability properties. Polym Degrad Stab 92: 1813-1823.
- RS Plentz, M Miotto, EE Schneider, MMC Forte, RS Mauler, et al. (2006) Effect of a macromolecular coupling agent on the properties of aluminum hydroxide/PP composites. J Appl Polym Sci 101: 1799-1805.
- Y Xie, CAS Hill, Z Xiao, H Militz, C Mai (2010) Silane coupling agents used for natural fiber/polymer composites: A review. Compos Part A: Appl Sci Manuf 41: 806-819.
- N Bouazizi, F Ajala, A Bettaibi, M Khelil, A Benghnia, et al. (2016) Metal-organo-zinc oxide materials: Investigation on the structural, optical and electrical properties. J Alloys Compd 656: 146-153.
- N Bouazizi, M Khelil, F Ajala, T Boudharaa, A Benghnia, et al. (2016) Molybdenum-loaded 1,5-diaminonaphthalene/ZnO materials with improved electrical properties and affinity towards hydrogen at ambient conditions. Int J Hydro Energy 41: 11232-11241.
- YX Wei, C Deng, ZY Zhao, YZ Wang (2018) A novel organic inorganic hybrid SiO2@DPP for the fire retardance of polycarbonate. Polym Degrad Stab 154: 177-185.
- D Wang, L Song, K Zhou, X Yu, Y Hu, et al. (2015) Anomalous nano-barrier effects of ultrathin molybdenum disulfide nanosheets for improving the flame retardance of polymer nanocomposites. J Mater Chem A 3: 14307-14317.
- MN Morshed, X Shen, H Deb, SA Azad, X Zhang, et al. (2018) Sonochemical fabrication of nanocryatalline titanium dioxide (TiO2) in cotton fiber for durable ultraviolet resistance. J Nat Fibers 1-14.
- UMA Khan, A Mariko, RJ Orlando (2017) Hybrid films of chitosan, cellulose nanofibrils and boric acid: Flame retardancy, optical and thermo-mechanical properties. Carbohydrate polymers 177: 13-21.
- Z Sheng, L Xiaodong, J Xiaodong, H Li, J Sun, et al. (2018) The novel application of chitosan: Effects of cross-linked chitosan on the fire performance of thermoplastic polyurethane. Carbohydrate Polymers 189: 313-321.
- L Zhong, H Yi-Ting, G Jin-Ping, RC Tang, GQ Chen (2019) Preparation of a flame retardant, antibacterial, and colored silk fabric with chitosan and vitamin B2 sodium phosphate by electrostatic layer by layer assembly. Materials Letters 241: 136-139.
- H Mohamed, N Mohamed, A Yasser, A Abdelkhali (2016) Synergistic effect of chitosan-based flame retardant and modified clay on the flammability properties of LLDPE. Polymer Degradation and Stability 133: 8-15.
- L Marcus, AOA Anas, RC Sarah, JC Grunlan (2015) Water-based chitosan/melamine polyphosphate multilayer nanocoating that extinguishes fire on polyester-cotton fabric. Carbohydrate polymers 130: 227-232.
- B Nabil, A Ahmed, G Stéphane, AE Achari, C Campagne, et al. (2020) Development of new composite fibers with excellent UV radiation protection. Physica E: Low-dimensional Systems and Nanostructures 118: 113905.
- PD Gajanan, PH Aravind, J Sushilkumar A, CR Patil, PS Patil (2019) A new method to prepare superhydrophobic cotton fabrics by post-coating surface modification of ZnO nanoparticles. Materials Letters 255: 126562.
- N Bouazizi, C Campagne, J Vieillard, V Julien, A Abdelkrim (2018) Polyfunctional cotton fabrics with catalytic activity and antibacterial capacity. Chemical Engineering Journal 351: 328-339.
- V Takke, N Behary, A Perwuelz, C Campagne (2009) Studies on the atmospheric air-plasma treatment of PET (polyethylene terephtalate) woven fabrics: Effect of process parameters and of aging. J Appl Polym Sci 114: 348-357.
- HB Ahmed, HE Emam (2016) Layer by layer assembly of nanosilver for high performance cotton fabrics. Fiber Polym 17: 418-426.
- HE Emam, M Rehan, HM Mashaly, HB Ahmed (2016) Large scaled strategy for natural/synthetic fabrics functionalization via immediate assembly of AgNPs. Dyes Pigm 133: 173-183.
- N Bachan, A Asha, WJ Jeyarani, DA Kumar, JM Shyla (2015) A comparative investigation on the structural, optical and electrical properties of SiO2-Fe3O4 Core-Shell nanostructures with their single components. Acta Metall Sinica 28: 1317-1325.
- F Ali, SB Khan, T Kamal, KA Alamry, EM Bakhsh, et al. (2018) Synthesis and characterization of metal nanoparticles templated chitosan-SiO2 catalyst for the reduction of nitrophenols and dyes. Carbohydr Polym 192: 217-230.
- B Lansk, GG Makarov, J Sebenda (1990) Termination reaction in the chain oxidation of caprolactam. Angew Makromol Chem 181: 143-152.
- G Pieter, D Weifu, Q Adam, M Celina (2016) Influence of temperature and stabilization on oxygen diffusion limited oxidation profiles of polyamide6. Polymer Degradation and Stability 130: 83-96.
- B Lansk (1994) Thermooxidation of lactam-based polyamides with carboxylic end-groups. Decomposition of 6-hydroperoxy-6-hexanelactam in the presence of carboxylic acids. Eur Polym J 30: 197-204.
- AT Hagler, A Lapiccirella (1976) Spatial electron distribution and population analysis of amides, carboxylic acids and peptides. Biopolymers 15: 1167-1200.
- AS Fomenko, TM Abramova, EP Dareva, AA Beloded, JG Furman (1968) Irradiation-chemical conversion of polyamide 6 and some aliphatic amides. Vysokomol Soedin A10: 507-514.
- A Kanakaraj, M Ashesh, SK Nataraj (2018) Electrospun nanofibers, nano composites and characterization of art: Insight on establishing fibers as product. Nanostructures & Nano-Objects 16: 45-58.
Author Details
Nabil Bouazizi1,2*, Ahmed Abed1,2,3, Stéphane Giraud1, Ahmida El Achari1, Christine Campagne1, Julien Vieillerd2 and Franck Le Derf2
1ENSAIT, GEMTEX - Laboratoire de Génie et Matériaux Textiles, France
2Normandie Univ., UNIROUEN, INSA Rouen, CNRS, France
3Laboratory LPMC, Faculty of Science El Jadida, Chouaib Doukkali University, Morocco
4Laboratory REMTEX, ESITH, Route d’El Jadida, Morocco
Corresponding author
Bouazizi Nabil, ENSAIT, GEMTEX - Laboratoire de Génie et Matériaux Textiles, F-59000, Lille, France; Normandie Univ., UNIROUEN, INSA Rouen, CNRS, COBRA (UMR 6014), 55 rue Saint Germain, 27000, Evreux, France.
Accepted: November 07, 2020 | Published Online: November 09, 2020
Citation: Bouazizi N, Abed A, Giraud S, El Achari A, Campagne C, et al. (2020) Polymers and TiO2 Based Nanocharges for Bio-Based Composites with Improved Flame Retardancy and Thermal Degradation. Int J Nanoparticles Nanotech 6:037.
Copyright: © 2020 Bouazizi N, et al. This is an open-access article distributed under the terms of the Creative Commons Attribution License, which permits unrestricted use, distribution, and reproduction in any medium, provided the original author and source are credited.
Abstract
The main objective of this work concerns the development of new eco-friendly composites based on biobased polymers for flame retardancy. Biobased cotton were employed as support for the fixation of biopolymers like chitosan (CT), titanium dioxide (TiO2) as nanocharges, and polyvinylidene fluoride (PVDF) as binder. The resulting composites CO-TiO2-PVDF-CT were thermally pressed to form compacted to ensure the surface compatibility. CO-TiO2-PVDF-CT were fully characterized by X-Ray diffractometer, scanning electron microscopy, optical microscopy, contact angle, Fourier transform IR spectroscopy and differential scanning calorimetry. The thermal degradation, chemical coatings, surface modification properties were investigated. It was found that CO-TiO2-PVDF-CT composite are highly hydrophobic materials with good diffusion resistance. PVDF and CT polymers induced an important role on the improvement of both flame retardancy and the thermal degradation. The degradation and elimination of FR from the composite product were studied. Finally, the toxicity of the prepared composite was performed to analyze the environmental impacts before and after the eco-path disposal treatment.
Keywords
Flame retardancy, Polymers, Nanocharges, Thermal degradation, Surface functionalization
Introduction
The flame retardancy (FR) of polymers or hybrid polymers and mineral matrix composites is conventionally produced by the dispersion of mineral fillers or retardant additives, especially based on halogenated compounds [1-3]. Most of these compounds were not efficient and present serious danger for both environment and humans. The majority of FR have adverse health and environmental effects from their exposure. Recently, researcher has investigated the concentrations of some FR in humans and the environment, which increased, because of their rapid and developed presence in our surroundings [4]. Their occurrences were highly increased in the manufacturing, use, and the disposal steps of the FR products. The exposure in humans occurs via ingestion of house dust and food, inhalation air, and thermal absorption [5]. Moreover, some works with regard to exposure of FR also induce exposure around disposal sites. In this promising prospect, a great attention has been given in developing novel FR products and alternates to toxic FR [6]. Unfortunately, few focusing and improving the end-of-life phase of FR textiles was found for in-depth analysis. In most of the studies, a comparative analysis is found on the environmental impact values for the manufacturing phase and use phase and frequently the manufacturing phase impact values are higher in synthetic fibers, while the use phase values are higher for the cotton fibers [7]. Consequently, a comparative analysis of end-of-life phase is urgently needed. In this regard, non-halogenated systems have been developed like compounds based on phosphorus, nitrogen or inorganic compounds. However the type these additives and the incorporation rate lead to the decreases the mechanical properties, but also degraded process ability [8]. In this context, intensive researches have been focused on developing advanced composites polymers with improved surface properties by using these metallic oxides. Abed, et al. have found that adding ZnO and SiO2 accelerates the carbonization of chlorinated poly (vinyl chloride) microfibers [8]. Metallic oxide were activated and modified by the addition of some active agent such as amine derivate for enhancing electrical and sensing properties or polyvinylidene fluoride (PVDF) to increase the surface area and the adsorption properties [8-13]. Due to their excellent flame retardancy, chemical and ecofriendly properties, polyvinylidene fluoride were widely used various applications such as the microfiltration and in advanced water separation and environmental remediation processes. Thus, composites based PVDF and MOx provided high thermal stability, flame retardancy properties, and impact resistance. This was explained by the presence of PVDF which incorporate metallic oxide, but also by the possible crosslink with the material [14-16]. To ensure the surface compatibility and the solid crosslinking between the composite species, researchers have employed some natural polymers as chitosan which show many advantages over artificial materials [17]. Recently, the chitosan was established as a good candidate in total biodegradability, biocompatibility with plants, antimicrobial, non-toxicity and fiber formation properties. Thus, chitosan is one of the most prospective materials for many fields as wastewater treatment, pulp and paper, agriculture, and textile fields [18-21].
To the best of our knowledge, studies relating a combination of all these FR additives have not been reported.
In this work, an alternative strategy to the functionalization and development of new composite based on polymers and biobased cotton fiber as ternary system for flame retardancy (Scheme 1). The methodology is based on the in-situ surface functionalization of biomaterials by using novel strategies to produce an effect if composite as flame retardancy. The surface compatibility, the thermal degradation and the life cycle assessment of the obtained composite were investigated in details. At last, the toxicity of the composite, which intends to verify the environmental benefits of proposed eco-path.
Materials and Methods
Chemicals
Natural chitosan (CT) as biopolymers, poly (vinylidene fluoride) (PVDF) and titanium dioxide TiO2 were purchased from Sigma-Aldrich. Acetone and N, N-dimethylformamide (DMF), ethanol, sodium triphosphate (PPT), distilled water, ammonia hydroxide (NH4OH, NH3 basis), sodium hydroxide (NaOH) were purchased and used as obtained. Biobased cotton fiber were purchased from Subrenat (Ref. UB1-FROM/ CR1.2).
Preparation and functionalization of biobased cotton
Scheme 1 showed the functionalization and design strategies employed for composite preparation. The process mainly consisted of the following steps: (i) The cotton fiber were cleaned to remove oil impurity, according our previously published works [22], (ii) PVDF and TiO2 were mixed in acetone and DMF (45:5 v/v) as solvent, and then stirred stirred during 2 h at 50 ℃. Upon the completely dissociation of TiO2, (iii) CT fibers were incubated in the obtained solution for 2 hours. The coated cotton materials were taken out from the solution and dried in at 50 ℃ for 24 h to clean and remove residual solvent. The obtained resulting composite named CO-PVDF-TiO2, were, then, prone to the chemical immobilization of chitosan (CT) by padding via an aqueous solution of 3 g/L chitosan biopolymer in presence of sodium triphosphate (PPT). The final prepared composites CO-PVDF-TiO2-CT were then dried at 110 ℃ during 3 min and cured at 170 ℃ for 30 second using a hot air dryer (Werner Mathis AG, Switzerland). Before the use for application and characterization, the composites were was hot-pressed (Scheme 2).
Characterizations
The prepared composite were fully characterized in this work. Fourier transform IR spectroscopy was carried out using a Tensor 27 (Bruker) spectrometer with a ZnSe ATR crystal. The samples were analyzed by FTIR directly without any further preparation, and background spectra were recorded on air. For field emission scanning electron microscopy (SEM) using a JEOL JEM-ARM200F HR setting with a field emission gun and probe aberration corrector, materials were previously metallized by a gold layer at 18 mA for 360 s with a Biorad E5200 device, and EDX images to evaluate the chemical contrast. Thermal analysis was measured by thermo gravimetric analysis (TG-DTA, A6300R). The water contact angle measurements were carried out by using Digi drop device from GBX. This instrument uses a goniometric method to calculate the contact angle between water and a solid surface.
Results and Discussions
Structural properties
The surface morphology of functionalized composites were obtained by SEM analysis, and the results are shown in Figure 1. The original cotton fiber exhibits a non-uniform network of overlapped fibers and multiple connected pores with a fairly smooth surface. The size of the fiber was changed between 21 μm and 15 μm and a visible void were observed in all the surface. After the functionalization steps, the fiber of the composites appeared linked the one with the other. This behavior can explained the successful coating steps by the addition of PVDF, CT and TiO2. The microstructure presented a continuous film structure with a thickness layer about few microns, forming a solid compacted surface. Herein, the key role of the functionalization strategy is the coherence and the compatibility of the compounds that employed during the preparation steps. This was supported by the totally covered fiber by the PVDF/TiO2/CT particles. Regarding to the total covered biobased cotton, it was supposed that a strong interfacial interaction between CO, PVDF, TiO2 and CT are involved. From optical and SEM images, it is clear that uniform distribution with high particles density were immobilized at the fiber surface. This was resulted in a much smoother surface structure and the formation of bulkier clusters of much smaller particles. Consequently, these changes can be explained in terms of strong interaction between CO, PVDF, TiO2 and CT, which promotes structure morphology with tailored surface.
Another confirmation was obtained from optical microscopy, indicating the good preponderance of uniform aggregates film at the fiber surface. Therefore, it appeared that both PDFV-MOx and CT successfully covered the PET fiber, in agreement with FTIR analysis, contact angle and TGA analysis.
Hydrophobic properties
Wettability and diffusion resistance are of great importance to evaluate the obtained CO-PVDF-TiO2-CT composite for environmental application. The contact angle and capillarity of the fiber surface were investigated and the results are reported in Figure 2. As seen, the water contact angle of original CO and CO-PVDF-TiO2-CT are 1.97 and 115, respectively. This result indicated that functionalized CO was highly hydrophobic. This result was of great importance, as composite with good hydrophobic characters would be beneficial for the enhancement not only the fire retardancy properties, but also the durability. Deeper insights in the changes of the apparent contact angles with time were investigated, and the results are summarized in Table 1. According to the obtained results, it was established that PVDF/TiO2/CT are responsible for the hydrophobic characters, as compared to the starting samples. Even, during 1 hour, the water cannot penetrate in CO-PVDF-TiO2-CT samples, due to the high hydrophobicity of PVDF and CT polymers. In our case, some unsaturated atoms like F from PVDF, Ti from TiO2, and N from CT can be increased this hyper hydrophobicity, as supported by the measurements on the contact angle. Herein, the role of chitosan was to ensure the crosslink between unsaturated atoms and hydroxyl groups from chitosan. Thus, the hydrophobicity was slightly increased by the CT grafting. This was confirmed by the droplet volume and the contact angle values, which were unchanged in time.
Infrared analysis
Infrared studies were carried out to ascertain the purity and nature of the functional groups that exhibited on prepared composites. The FTIR spectra of original and modified CO nonwovens are shown in Figure 3. The structure of original cotton displayed some bands in the region 1720-650 cm-1, attributed to the stretching vibration of CH2, C=O, and aromatic C=C. The peaks obtained between 900 and 1285 cm-1 corresponded to asymmetric out-of-phase ring stretch: C1-O-C4 glucosidic bond, 1030 to 1031 cm-1 C-O stretching and 1280 to 1285 cm-1 due to C-H deformation stretch [23]. This was in agreement with the XRD Figure 4 which revealed the characteristic peaks of cellulose at 2 Θ =23° and 30° [24].
The broad absorption peaks at around 3437 and 1456 cm-1 are attributed to stretching vibrations of OH groups corresponding to the adsorbed water molecules on the samples surface. After PVDF fixation, the bands obtained at 869 cm-1 were attributed to the vibrations of υ CF2 for amorphous PVDF. The impregnation of TiO2 nanocharges was confirmed by the band at 700 cm-1 assigned to the stretching vibration of Ti-O. Chitosan grafting induced a shift of the υ CF2 peaks from 869 cm-1 to 875 cm-1 which might be due to the restriction on the vibration by the interaction occurred between TiO2, CT and CF2 groups. The peak obtained at 1625 cm-1 was assigned to the NH2 group bend scissoring, indicating that chitosan were successfully coated the modified cotton fibers [25-29].
Thermogravimetric analysis
The TGA curves of all samples are shown in Figure 5. From this analysis, two different stages are appeared in the range of 380 ℃ and 400 ℃, indicating the decomposition of the composites. As seen the maximum decomposition peak are obtained at 423 ℃ for CO-TiO2-PVDF-CT and towards 435 ℃ for CO-TiO2-PVDF composites. In addition, around 525 ℃ for the two composites, a partial decomposition were displayed, which attributed to the formation of residues or oxidation of volatiles species [22,24]. By comparing the analysis in atmosphere and nitrogen, it is clear that decomposition of the composite is favored due to the absence of oxygen to oxidize. However, for the other intermediates composites the intensity peak is much lower. This result can be explained that TGA under nitrogen evidenced the presence of some substances consisting mainly of cyclic monomer or hemicellulose from the cotton materials. There is a mass increment process for CO-PVDF-TiO2-CT at the range of 300-400 ℃, indicating the effect of TiO2 on the thermal properties Table 2. Here, the initial decomposition temperature of CO and CO-PVDF-TiO2-CT are 333 ℃ and 480 ℃, respectively. The high temperature at maximum weight loss rate can also be found for these hybrids and the solid residues CO-PVDF-TiO2 at 600 ℃ are 84.5%, respectively, demonstrating again their good thermal stability, particularly for CO-PVDF-TiO2 which confirms again the good dispersion of TiO2 nanocharge.
Flame retardancy of composites
Flame-retardant performances of the as-prepared CO-PVDF-TiO2-CT composites were studied and reported in Figure 6. The first overview demonstrates that original biobased material was a highly flammable, and the surface was completely burned. However, the functionalized CO displayed a visible improvement in the FR rate. The combustion time was increased for the treated samples from 30 second for pristine CO to 1.3 minutes for CO-PVDF-TiO2-CT Table 3. This important signal evidenced the key role of our functionalization steps. Hence, this result can be explained by the combination between polymers (CT and PVDF) with nanocharges (TiO2) that decrease the weight loss and keep the organization of the fiber structure and the skeleton. Therefore, the PVDF/TiO2/CT combination act as an efficient organic-inorganic material, playing the role of barrier to limit and slowing the fire spread, and thus the resistances to fire growth were improved. Results on CO-PVDF-TiO2-CT present good properties on FR because of the reduction of the fire spread and the time of burned are highly reduced.
Smoke propagation
Regarding to the images obtained after FR test, the residues amount of CO-PVDF-TiO2-CT were higher, which indicate that corresponding composite was of great performance of char production. Here, a confirmation was obtained by the smoke release during the composite combustion. No visible smoke can be obtained during the combustion process, suggesting that prepared CO-PVDF-TiO2-CT composites released little smoke gas. However, it was found that fiber surface was completely coated by char, which may explain the adsorption of smoke at the composite surface without releases. Here, the smoke formation on the surface fiber was accompanied by an improvement on the flame-retardant by the creation of char layer. As expected, only CO-PVDF-TiO2-CT presents a visible smoke as compared to the other materials, indicating that chitosan was a good candidate to catalyze FR. This was reflected by the CT/PVDF contained flame retardants, which can interact with free radicals and then limit the flammability of the composites [24]. These results were confirmed by the clear decreases of destroyed area Table 3. Therefore, the difference of char formation and the obtained residues among the addition of chemicals elements can visually demonstrate the key role of the post modification into the biobased cotton in their FR.
Effect of the functionalization steps on the FR
The surface modification and comparisons between the different functionalization steps were studied on the bases of FR results. It can be observed that CO-PVDF-TiO2-CT composite have affinity towards the FR, and a variety of char residue was obtained Figure 7. This was an additional evidence for the successful chemical treatments on PET. The amount of char residue was little for CO-PVDF-TiO2-CT composite, suggesting that nano charge produced more char residue, as supported by the visible agglomerated particles at the fiber surface. This result is of great importance, because it provides clear evidence of the efficiency of TiO2 nanocharge on the formation of char residue and on FR. Additional, attempts for investigating the role of the above chemical elements were achieved with CO-PVDF-TiO2-CT composite. Here, the results show high improvement of the FR, that induced by the well route employed for the synthesis of the novel polymer functional composite. The improvement on FR resulted from CO-PVDF-TiO2-CT composite must be due to the high capacity of Ti atoms to diffuse or to promote Lewis acid-base interactions with the surface polymers (i.e. CT and PVDF). A possible explanation resides in the formation of carbonates and/or carbonate-like associations with traces of nanocharge and the surrounding lattice oxygen atoms. This must involve multiple interactions and agrees with the significant enhancement of the hydrophobic character, as confirmed by the contact angle measurements (Scheme 3).
Thermal degradation mechanism of polymers
As known, to identify the links between the polymers, three activated sites can be involved on the as-prepared composite CO-TiO2-PVDF-CT. These candidates are the amide bond, the carbon-carbon bond in the segment -CH2-CO of CT. As with CO-TiO2-PVDF-CT, a process intramolecular and hydrogen transfer leading to the cleavage of the C-N bond were identified [30]. Radicals which result there from are able for forming carbon monoxide for the composite. According to Gijsman, et al. [31], the main degradation of composites can be illustrated by a mechanism of cyclic degradation. Firstly, the formation of polymeric chain with the cotton fiber as a group in end of composite. Secondly, an equilibrium reaction is involved between this polymeric chain and the cotton fiber. Finally, the primary decomposition process is that involving a hydrogen-C-H to nitrogen (N-H) transfer reaction [32-34]. This reaction leading to scission of the chain and the evolution of the fragments terminated by chitosan units. These facts is related to the correlation between the amount of formed polymeric chain and the hydrogen bond fraction in polyamides [31]. Indeed, the connections hydrogen cause crosslinking by keeping the polymeric chains close during the degradation. The hydrogen bond can lead to an abstraction of hydrogen alongside nitrogen, which leads to crosslinking and the formation of a structure pyrrole-based conjugate. The nanocharges are regularly dispersed with, however, a slight tendency to aggregate. However, it has been observed that fibers of the CO fiber support have been well coated by the layer of the composite CO-PVDF- TiO2-CT. This can explain the creation of a very strong interphase bond between the surface of the molecules of metal oxides and the polymer chains (Scheme 3).
Effect of nanocharges on the flame retardancy
Nanocharges based on TiO2 are responsible for slowing highly the combustion of any materials, throughout inhibition of the radical flame propagation reactions in the gas phase. Indeed, the active radicals HO• can be adsorbed on the surface of the oxide particles. Therefore, collision energy is transferred to oxides and then less reactive HOO• radicals are formed in comparison to those of initial HO•. Aruchamy, et al. [35] proposed that improving the thermal stability of composites could be explained by the hydrogen bonding interaction between the carbonyl groups and the hydroxyl groups present on the surface of the composite. Consequently, hydrogen bonds induced a visible slowing on the depolymerization of the polymer chains. Herein, this improvement of the FR was explained by an effect of electrons capture by nanocharges particles, which act as a gas barrier, preventing the diffusion of degradation products outside the composite (Scheme 4).
Toxicity of the composite
Polyvinylidene fluoride has achieved a superior degree of employment upon good resistance to chemicals, and oxidants and fire retardancy. Herein, the toxicity of CO-PVDF-TiO2-CT has been studied using COD technique of the solution after washing process. Results demonstrated that COD removals increased from 0% to 81% for CO and CO-PVDF-TiO2-CT, respectively. The main reason for this tendency may due to the decreases number of organic molecules, particularly with the adding of CO-PVDF-TiO2-CT composite. Consequently, the gas suppression is due to the generation of activated site whereas highly efficient to adsorb the gas in the fibers surface. This was confirmed by the highly decreases of oxygens, as supported by the FR tests.
Conclusions
Regarding to the high demand for research in the field of polymers composites with improved flame retardancy, this work explored an overview of the preparation, functionalization and application of polymer composite based on the combination between biobased cotton (CO), polyvinylidene fluoride (PVDF), chitosan (CT) and nanocharge (TiO2). It was found that FR were highly improved after the addition of PVDF, TiO2 and CT compounds. The good thermal degradation of the prepared composite evidenced the key role of the functionalization strategy. Regarding to the above results, our functionalization approach can be used in several applications such textile for fire retardancy and environment protection. Thus, new advanced ways for further research for both human and environment safety can be opened.